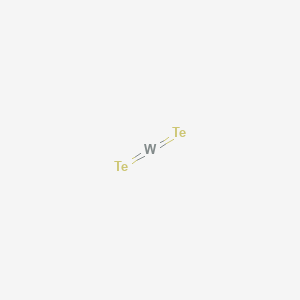
Tungsten telluride (WTe2)
Overview
Description
Tungsten telluride (WTe₂) is a layered transition metal dichalcogenide (TMD) with an orthorhombic crystal structure (Td phase), where tungsten atoms form quasi-1D chains along the a-axis, sandwiched between distorted tellurium layers . Unlike most TMDs (e.g., MoS₂, WS₂), which are semiconductors, WTe₂ exhibits semimetallic behavior with a small Fermi surface composed of electron and hole pockets . Its unique electronic structure enables extraordinary properties:
- Extreme Magnetoresistance (XMR): WTe₂ shows a 13-million% increase in resistivity at 0.5 K under a 60 T magnetic field, attributed to electron-hole compensation and topological protection .
- Type-II Weyl Semimetal Behavior: Bulk WTe₂ hosts Weyl fermions with tilted Dirac cones, leading to anisotropic electronic responses .
- Pressure-Induced Superconductivity: Above 10.5 GPa, WTe₂ transitions to a superconductor with a critical temperature (Tc) of 6.5 K at 13 GPa .
- Layer-Dependent Insulating State: Monolayer WTe₂ becomes a robust insulator, yet exhibits quantum oscillations under magnetic fields—a paradox challenging existing theories .
Preparation Methods
Synthetic Routes and Reaction Conditions: The synthesis of TNP-2092 involves the covalent bonding of rifamycin with a quinolizinone pharmacophore. The process includes multiple steps of organic synthesis, such as esterification, amidation, and cyclization reactions. Specific reaction conditions, such as temperature, pH, and solvent choice, are optimized to ensure high yield and purity of the final product .
Industrial Production Methods: Industrial production of TNP-2092 follows a similar synthetic route but is scaled up to meet commercial demands. The process involves large-scale reactors and stringent quality control measures to ensure consistency and compliance with regulatory standards. The production is carried out in Good Manufacturing Practice (GMP) certified facilities to ensure the safety and efficacy of the drug .
Chemical Reactions Analysis
Bromine-Assisted CVT
-
Process : Stoichiometric mixtures of tungsten (W) and tellurium (Te) powders are sealed in evacuated quartz ampoules with bromine (Br₂) as the transport agent (~6 mg/cm³) .
-
Temperature Gradient : A two-zone furnace maintains temperatures between 775°C (growth zone) and 950°C (reaction zone) for 4–5 days .
-
Outcome : Platelet-shaped Td-WTe₂ crystals (orthorhombic structure) with a W:Te ratio of 1:1.9, indicating slight Te deficiency .
Tellurium Tetrachloride (TeCl₄) CVT
-
Alternative Method : TeCl₄ serves as the transport agent in a similar temperature range (1073–1173 K), yielding large crystals with high crystallinity .
Oxidation and Environmental Reactivity
WTe₂ exhibits significant sensitivity to ambient conditions:
-
Surface Oxidation : Prolonged air exposure leads to surface oxidation, forming WO₃ and TeO₂ .
-
Impact on Applications : Oxidation degrades electronic properties, necessitating inert storage or protective coatings for device integration .
Thermal Decomposition and Stability
WTe₂ undergoes decomposition under high-temperature or reactive environments:
Condition | Reaction Outcome | Reference |
---|---|---|
>750°C in H₂ atmosphere | Decomposes to elemental W | |
Prolonged heating at 800°C | Forms WO₃ and Te vapors |
Thermodynamic Stability :
-
The Td phase (distorted 1T structure) is thermodynamically favored over the 2H phase by 0.58 eV per formula unit at equilibrium .
High-Pressure Reactions
Under extreme pressure, WTe₂ transitions to superconducting states:
-
Pressure Threshold : >10.5 GPa induces superconductivity below 6.5 K .
-
Mechanism : Suppression of magnetoresistance and structural phase transitions under compression .
Reaction with Transport Agents
Residual bromine or TeCl₄ from synthesis requires post-growth purification:
-
Dynamic Vacuum Treatment : Removes trapped Br₂ or TeCl₄ from crystal surfaces .
-
Surface Cleaning : Sequential immersion in acetone and isopropanol eliminates contaminants .
Thin-Film Growth Reactions
Chemical Vapor Deposition (CVD) :
-
Precursor : Tungsten hexacarbonyl (W(CO)₆) reacts with Te vapors at 600°C, forming oriented Td-WTe₂ films .
-
Substrate Influence : Quartz and sapphire substrates yield nanocrystalline films with low thermal conductivity (~1.2 W/m·K) .
Key Research Findings
-
Metallic Nature : Td-WTe₂ exhibits semimetallic behavior with 0.21 eV indirect band overlap (Γ to X direction) .
-
Anisotropic Reactivity : Oxidation and intercalation occur preferentially along the a-axis due to zigzag W chains .
-
Magnetoresistance : WTe₂ shows a 13 million% resistance increase at 60 T and 0.5 K, linked to compensated electron-hole populations .
Table 2: Thermal Decomposition Pathways
Condition | Products | Application Impact |
---|---|---|
>750°C in H₂ | W + Te vapors | Film instability |
Ambient oxidation | WO₃ + TeO₂ | Device degradation |
This synthesis of experimental and theoretical data underscores WTe₂’s reactivity under thermal, chemical, and pressure stimuli, informing its use in next-generation electronics and quantum materials .
Scientific Research Applications
Electronic Applications
Weyl Semimetal Properties
WTe2 is recognized as a type-II Weyl semimetal, which allows it to exhibit unique electronic transport properties, including large non-saturating magnetoresistance. This characteristic makes it suitable for applications in advanced electronic devices, such as:
- Magnetic Sensors : The high magnetoresistance observed in WTe2 can be exploited in the development of sensitive magnetic field sensors. Research has demonstrated that doping WTe2 with magnetic ions can induce magnetic ordering, enhancing its potential for sensor applications .
- Field-Effect Transistors : The anisotropic electrical properties of WTe2 make it a candidate for field-effect transistors (FETs). The ability to manipulate its electronic properties through mechanical exfoliation and layer thickness variation allows for the design of high-performance FETs .
Data Table: Electronic Properties of WTe2
Property | Value |
---|---|
Type | Type-II Weyl Semimetal |
Magnetoresistance | Non-saturating up to 60 T |
Carrier Mobility | High (specific values vary) |
Anisotropy Ratio | 1.89 (zigzag/armchair) |
Thermoelectric Applications
WTe2 has been identified as an excellent thermoelectric material due to its high thermoelectric efficiency. Its ability to convert temperature differences into electric voltage can be harnessed in various applications:
- Thermoelectric Generators : The high Seebeck coefficient and low thermal conductivity of WTe2 make it ideal for thermoelectric generators that convert waste heat into usable electrical energy. Recent studies have shown that multilayer WTe2 exhibits improved thermal anisotropy, enhancing its thermoelectric performance .
- Cooling Devices : The unique thermal properties of WTe2 can also be utilized in cooling applications, where it can serve as a solid-state cooling material.
Data Table: Thermoelectric Properties of WTe2
Property | Value |
---|---|
Seebeck Coefficient | High (specific values vary) |
Thermal Conductivity | Low |
Figure of Merit | High (specific values vary) |
Quantum Material Applications
WTe2's distinctive quantum properties open avenues for research in quantum computing and advanced materials science:
- Quantum Computing : The topological nature of WTe2 allows for the exploration of new quantum states of matter, which could lead to advancements in quantum computing technologies. Research has shown that twisted bilayer structures of WTe2 can host one-dimensional physics, potentially paving the way for novel quantum computational architectures .
- Superconductivity Studies : Under high-pressure conditions, WTe2 exhibits superconductivity, providing a platform for studying superconducting phenomena in transition metal dichalcogenides. This property is particularly relevant for developing next-generation superconducting devices .
Case Study: Superconductivity in WTe2
In experiments, superconductivity was induced in WTe2 at pressures above 2.5 GPa, reaching a maximum critical temperature of 7 K at approximately 16.8 GPa. This behavior illustrates the material's potential for superconducting applications under varying environmental conditions .
Mechanism of Action
TNP-2092 exerts its antibacterial effects by inhibiting three key bacterial enzymes: RNA polymerase, DNA gyrase, and topoisomerase IV. This multi-targeted approach disrupts bacterial DNA replication, transcription, and repair processes, leading to bacterial cell death. The compound’s dual-acting nature reduces the likelihood of resistance development, making it a promising candidate for treating persistent infections .
Comparison with Similar Compounds
Comparison with Similar Compounds
Crystal Structure and Phase Stability
WTe₂’s Td phase arises from Peierls distortion, absent in MoTe₂ and other TMDs, leading to its distinct metallic behavior .
Electronic Properties
WTe₂’s semimetallic nature contrasts with the semiconducting behavior of WSe₂ and WS₂. MoTe₂ shares some topological features but requires precise phase engineering .
Electrical and Magnetic Properties
WTe₂’s XMR outperforms MoTe₂ and is absent in WSe₂. Its pressure-induced superconductivity is distinct from MoTe₂’s ambient-pressure instability .
Data Tables
Table 1: Physical Properties of Select TMDs
Material | Density (g/cm³) | Melting Point (°C) | Magnetoresistance (%) | Thermal Conductivity (W/m·K) |
---|---|---|---|---|
WTe₂ | 9.43 | 1020 | 13,000,000 | 5–10 |
MoTe₂ | 7.7 | 790 | 500 | 3–5 |
WSe₂ | 9.2 | 1200 | Negligible | 20–30 |
Table 2: Electronic Transport Comparison
Material | Resistivity (Ω·cm) | Carrier Mobility (cm²/Vs) | Effective Mass (me) |
---|---|---|---|
WTe₂ | 10⁻³–10⁻⁴ | 10⁴–10⁵ | 0.1–0.3 |
MoTe₂ | 10⁻²–10⁻³ | 200–500 | 0.2–0.5 |
WSe₂ | 10⁻¹–10⁻² | 50–100 | 0.5–0.8 |
Biological Activity
Tungsten telluride (WTe2) is a transition metal dichalcogenide (TMD) that has garnered significant interest due to its unique electronic properties and potential applications in various fields, including electronics and materials science. Despite its promising characteristics, the biological activity of WTe2 remains underexplored. This article aims to provide a comprehensive overview of the biological implications of WTe2, supported by relevant data tables and case studies.
Overview of Tungsten Telluride (WTe2)
WTe2 exists in several structural forms, with the most studied being the distorted 1T structure (Td-WTe2). This structure exhibits metallic-like transport properties and demonstrates significant stability under various conditions. The compound has been shown to possess remarkable electronic properties, including a high magnetoresistance effect, which has implications for its use in magnetic sensors and other electronic devices .
Toxicity and Biocompatibility
Research on the biological activity of WTe2 primarily focuses on its toxicity and potential biocompatibility. Preliminary studies suggest that while WTe2 does not exhibit acute toxicity at low concentrations, its effects on cellular systems, particularly in prolonged exposure scenarios, require further investigation.
- Cellular Interactions : Initial studies indicate that WTe2 can interact with cellular membranes, potentially leading to cellular stress responses. The specific mechanisms through which WTe2 exerts these effects are still being elucidated.
- Oxidative Stress : Exposure to WTe2 has been associated with increased oxidative stress markers in certain cell lines, suggesting that it may induce reactive oxygen species (ROS) production. This is critical as ROS can lead to cellular damage and inflammation .
- Inflammatory Responses : Some studies have indicated that WTe2 may influence inflammatory pathways, although detailed mechanisms remain unclear. Understanding these interactions is crucial for assessing the safety of WTe2 in biomedical applications.
Case Studies
A few notable studies have explored the biological effects of WTe2:
- Study 1 : A study evaluated the cytotoxic effects of WTe2 nanoparticles on human lung epithelial cells. Results showed a dose-dependent increase in cell death at higher concentrations, highlighting the need for careful consideration of dosage in potential therapeutic applications .
- Study 2 : Another investigation focused on the immunological responses elicited by WTe2 exposure in murine models. The findings suggested that WTe2 could modulate immune responses, although further research is necessary to clarify these effects and their implications for human health .
Data Tables
The following table summarizes key findings from various studies regarding the biological activity of WTe2:
Q & A
Q. Basic: What are the optimal synthesis parameters for growing high-quality WTe₂ single crystals?
Answer:
High-quality WTe₂ crystals are typically synthesized via chemical vapor transport (CVT) using iodine as a transport agent. Optimal parameters include:
- Temperature gradient : 700°C (source) to 650°C (sink) for 7–10 days .
- Precursor stoichiometry : Tungsten and tellurium in a 1:2 molar ratio, with excess tellurium to compensate for volatility.
- Post-synthesis characterization : X-ray diffraction (XRD) for phase identification, transmission electron microscopy (TEM) for defect analysis, and energy-dispersive X-ray spectroscopy (EDS) for stoichiometric validation .
Q. Basic: How do structural defects in WTe₂ influence its electrical transport properties?
Answer:
Defects such as vacancies or grain boundaries can significantly alter carrier mobility and magnetoresistance. Methodologies include:
- Defect engineering : Controlled introduction of vacancies via annealing in tellurium-deficient environments.
- Transport measurements : Four-probe resistivity and Hall effect measurements under varying temperatures (2–300 K) to correlate defect density with carrier scattering .
Q. Advanced: How can conflicting reports on WTe₂’s topological surface states be resolved?
Answer:
Contradictions arise from sample quality and measurement conditions. A multi-technique approach is critical:
- Angle-resolved photoemission spectroscopy (ARPES) : Resolves surface vs. bulk contributions by probing electronic band structure under ultra-high vacuum.
- Scanning tunneling microscopy (STM) : Maps surface topography and local density of states at atomic resolution.
- Density functional theory (DFT) : Validates experimental observations by modeling defect-free surfaces .
Q. Advanced: What methodologies effectively probe the nonlinear optical response of WTe₂?
Answer:
WTe₂’s broken inversion symmetry enables strong second-harmonic generation (SHG). Key methods include:
- Pump-probe spectroscopy : Quantifies carrier dynamics and exciton lifetimes under femtosecond laser excitation.
- Polarization-resolved SHG : Maps crystal orientation and domain boundaries.
- Terahertz time-domain spectroscopy : Measures nonlinear conductivity in the THz regime, critical for optoelectronic applications .
Q. Basic: What are the challenges in achieving consistent stoichiometry in WTe₂ thin films?
Answer:
Stoichiometric drift during sputtering or chemical vapor deposition (CVD) is common. Mitigation strategies:
- In-situ monitoring : Use X-ray photoelectron spectroscopy (XPS) or Rutherford backscattering spectrometry (RBS) during growth.
- Post-deposition annealing : Optimize temperature (250–400°C) and chalcogen pressure to restore stoichiometry .
Q. Advanced: How does layer stacking configuration affect electronic correlations in WTe₂ heterostructures?
Answer:
Interlayer twist angles and stacking order modulate charge density waves and superconductivity. Approaches include:
- Molecular beam epitaxy (MBE) : Precision-controlled layer-by-layer growth.
- Cross-sectional STEM : Visualizes stacking sequences and interfacial defects.
- Magnetotransport studies : Identifies anomalies in quantum oscillations tied to stacking-induced symmetry breaking .
Q. Basic: Which characterization techniques are critical for confirming the crystal structure of WTe₂?
Answer:
- XRD : Confirms the orthorhombic (Td) phase via Bragg peak indexing.
- Raman spectroscopy : Detects phonon modes (e.g., A₁g and B₂g peaks) sensitive to layer thickness and strain.
- Selected-area electron diffraction (SAED) : Validates crystallographic orientation in TEM .
Q. Advanced: What experimental designs minimize environmental oxidation during WTe₂ device fabrication?
Answer:
WTe₂ oxidizes rapidly in air. Solutions include:
Properties
IUPAC Name |
bis(tellanylidene)tungsten | |
---|---|---|
Source | PubChem | |
URL | https://pubchem.ncbi.nlm.nih.gov | |
Description | Data deposited in or computed by PubChem | |
InChI |
InChI=1S/2Te.W | |
Source | PubChem | |
URL | https://pubchem.ncbi.nlm.nih.gov | |
Description | Data deposited in or computed by PubChem | |
InChI Key |
WFGOJOJMWHVMAP-UHFFFAOYSA-N | |
Source | PubChem | |
URL | https://pubchem.ncbi.nlm.nih.gov | |
Description | Data deposited in or computed by PubChem | |
Canonical SMILES |
[Te]=[W]=[Te] | |
Source | PubChem | |
URL | https://pubchem.ncbi.nlm.nih.gov | |
Description | Data deposited in or computed by PubChem | |
Molecular Formula |
WTe2, Te2W | |
Record name | tungsten(IV) telluride | |
Source | Wikipedia | |
URL | https://en.wikipedia.org/wiki/Tungsten(IV)_telluride | |
Description | Chemical information link to Wikipedia. | |
Source | PubChem | |
URL | https://pubchem.ncbi.nlm.nih.gov | |
Description | Data deposited in or computed by PubChem | |
DSSTOX Substance ID |
DTXSID3065243 | |
Record name | Tungsten telluride (WTe2) | |
Source | EPA DSSTox | |
URL | https://comptox.epa.gov/dashboard/DTXSID3065243 | |
Description | DSSTox provides a high quality public chemistry resource for supporting improved predictive toxicology. | |
Molecular Weight |
439.0 g/mol | |
Source | PubChem | |
URL | https://pubchem.ncbi.nlm.nih.gov | |
Description | Data deposited in or computed by PubChem | |
CAS No. |
12067-76-4 | |
Record name | Tungsten telluride (WTe2) | |
Source | CAS Common Chemistry | |
URL | https://commonchemistry.cas.org/detail?cas_rn=12067-76-4 | |
Description | CAS Common Chemistry is an open community resource for accessing chemical information. Nearly 500,000 chemical substances from CAS REGISTRY cover areas of community interest, including common and frequently regulated chemicals, and those relevant to high school and undergraduate chemistry classes. This chemical information, curated by our expert scientists, is provided in alignment with our mission as a division of the American Chemical Society. | |
Explanation | The data from CAS Common Chemistry is provided under a CC-BY-NC 4.0 license, unless otherwise stated. | |
Record name | Tungsten telluride (WTe2) | |
Source | ChemIDplus | |
URL | https://pubchem.ncbi.nlm.nih.gov/substance/?source=chemidplus&sourceid=0012067764 | |
Description | ChemIDplus is a free, web search system that provides access to the structure and nomenclature authority files used for the identification of chemical substances cited in National Library of Medicine (NLM) databases, including the TOXNET system. | |
Record name | Tungsten telluride (WTe2) | |
Source | EPA Chemicals under the TSCA | |
URL | https://www.epa.gov/chemicals-under-tsca | |
Description | EPA Chemicals under the Toxic Substances Control Act (TSCA) collection contains information on chemicals and their regulations under TSCA, including non-confidential content from the TSCA Chemical Substance Inventory and Chemical Data Reporting. | |
Record name | Tungsten telluride (WTe2) | |
Source | EPA DSSTox | |
URL | https://comptox.epa.gov/dashboard/DTXSID3065243 | |
Description | DSSTox provides a high quality public chemistry resource for supporting improved predictive toxicology. | |
Record name | Tungsten ditelluride | |
Source | European Chemicals Agency (ECHA) | |
URL | https://echa.europa.eu/substance-information/-/substanceinfo/100.031.884 | |
Description | The European Chemicals Agency (ECHA) is an agency of the European Union which is the driving force among regulatory authorities in implementing the EU's groundbreaking chemicals legislation for the benefit of human health and the environment as well as for innovation and competitiveness. | |
Explanation | Use of the information, documents and data from the ECHA website is subject to the terms and conditions of this Legal Notice, and subject to other binding limitations provided for under applicable law, the information, documents and data made available on the ECHA website may be reproduced, distributed and/or used, totally or in part, for non-commercial purposes provided that ECHA is acknowledged as the source: "Source: European Chemicals Agency, http://echa.europa.eu/". Such acknowledgement must be included in each copy of the material. ECHA permits and encourages organisations and individuals to create links to the ECHA website under the following cumulative conditions: Links can only be made to webpages that provide a link to the Legal Notice page. | |
Disclaimer and Information on In-Vitro Research Products
Please be aware that all articles and product information presented on BenchChem are intended solely for informational purposes. The products available for purchase on BenchChem are specifically designed for in-vitro studies, which are conducted outside of living organisms. In-vitro studies, derived from the Latin term "in glass," involve experiments performed in controlled laboratory settings using cells or tissues. It is important to note that these products are not categorized as medicines or drugs, and they have not received approval from the FDA for the prevention, treatment, or cure of any medical condition, ailment, or disease. We must emphasize that any form of bodily introduction of these products into humans or animals is strictly prohibited by law. It is essential to adhere to these guidelines to ensure compliance with legal and ethical standards in research and experimentation.