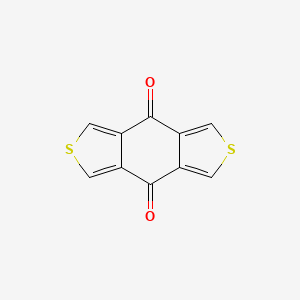
2,6-Dithia-s-indacene-4,8-dione
Overview
Description
2,6-Dithia-s-indacene-4,8-dione is an organic compound with the molecular formula C10H4O2S2 It is characterized by its unique structure, which includes two sulfur atoms and two carbonyl groups within a fused ring system
Preparation Methods
Synthetic Routes and Reaction Conditions: The synthesis of 2,6-Dithia-s-indacene-4,8-dione typically involves the cyclization of appropriate precursors under specific conditions. One common method involves the reaction of 2,5-dihydroxy-1,4-benzoquinone with sulfur-containing reagents. The reaction is usually carried out in the presence of a catalyst, such as a Lewis acid, under controlled temperature and pressure conditions to ensure the formation of the desired product.
Industrial Production Methods: Industrial production of this compound may involve similar synthetic routes but on a larger scale. The process is optimized for higher yields and purity, often involving continuous flow reactors and advanced purification techniques such as recrystallization and chromatography.
Chemical Reactions Analysis
Types of Reactions: 2,6-Dithia-s-indacene-4,8-dione undergoes various chemical reactions, including:
Oxidation: The compound can be oxidized to form sulfoxides or sulfones.
Reduction: Reduction reactions can convert the carbonyl groups to hydroxyl groups.
Substitution: The compound can participate in nucleophilic substitution reactions, where one of the sulfur atoms is replaced by another nucleophile.
Common Reagents and Conditions:
Oxidation: Common oxidizing agents include hydrogen peroxide and peracids.
Reduction: Reducing agents such as lithium aluminum hydride (LiAlH4) or sodium borohydride (NaBH4) are typically used.
Substitution: Nucleophiles like amines or thiols can be used under basic conditions to facilitate substitution reactions.
Major Products: The major products formed from these reactions depend on the specific reagents and conditions used. For example, oxidation can yield sulfoxides or sulfones, while reduction can produce diols.
Scientific Research Applications
2,6-Dithia-s-indacene-4,8-dione has a wide range of applications in scientific research:
Chemistry: It is used as a building block for the synthesis of more complex organic molecules and as a ligand in coordination chemistry.
Biology: The compound’s unique structure allows it to interact with biological macromolecules, making it useful in the study of enzyme mechanisms and protein-ligand interactions.
Medicine: Research is ongoing into its potential use as a therapeutic agent, particularly in the development of drugs targeting specific enzymes or receptors.
Industry: It is used in the production of advanced materials, such as conductive polymers and organic semiconductors.
Mechanism of Action
The mechanism by which 2,6-Dithia-s-indacene-4,8-dione exerts its effects is largely dependent on its interaction with molecular targets. The compound can act as an electron donor or acceptor, facilitating redox reactions. It can also form stable complexes with metal ions, influencing various biochemical pathways. The specific pathways involved depend on the context of its use, whether in a biological system or an industrial application.
Comparison with Similar Compounds
- 2,6-Dibromo-1,5-dithia-s-indacene-4,8-dione
- 1,3-Dichloro-2,6-dithia-s-indacene-4,8-dione
Comparison: Compared to these similar compounds, 2,6-Dithia-s-indacene-4,8-dione is unique due to its specific substitution pattern and the presence of two carbonyl groups. This structural difference imparts distinct chemical reactivity and physical properties, making it particularly useful in applications requiring specific electronic or steric characteristics.
Properties
IUPAC Name |
thieno[3,4-f][2]benzothiole-4,8-dione | |
---|---|---|
Details | Computed by Lexichem TK 2.7.0 (PubChem release 2021.05.07) | |
Source | PubChem | |
URL | https://pubchem.ncbi.nlm.nih.gov | |
Description | Data deposited in or computed by PubChem | |
InChI |
InChI=1S/C10H4O2S2/c11-9-5-1-13-2-6(5)10(12)8-4-14-3-7(8)9/h1-4H | |
Details | Computed by InChI 1.0.6 (PubChem release 2021.05.07) | |
Source | PubChem | |
URL | https://pubchem.ncbi.nlm.nih.gov | |
Description | Data deposited in or computed by PubChem | |
InChI Key |
YCQHVKQGWLAQMR-UHFFFAOYSA-N | |
Details | Computed by InChI 1.0.6 (PubChem release 2021.05.07) | |
Source | PubChem | |
URL | https://pubchem.ncbi.nlm.nih.gov | |
Description | Data deposited in or computed by PubChem | |
Canonical SMILES |
C1=C2C(=CS1)C(=O)C3=CSC=C3C2=O | |
Details | Computed by OEChem 2.3.0 (PubChem release 2021.05.07) | |
Source | PubChem | |
URL | https://pubchem.ncbi.nlm.nih.gov | |
Description | Data deposited in or computed by PubChem | |
Molecular Formula |
C10H4O2S2 | |
Details | Computed by PubChem 2.1 (PubChem release 2021.05.07) | |
Source | PubChem | |
URL | https://pubchem.ncbi.nlm.nih.gov | |
Description | Data deposited in or computed by PubChem | |
DSSTOX Substance ID |
DTXSID90187172 | |
Record name | 2,6-Dithia-s-indacene-4,8-dione | |
Source | EPA DSSTox | |
URL | https://comptox.epa.gov/dashboard/DTXSID90187172 | |
Description | DSSTox provides a high quality public chemistry resource for supporting improved predictive toxicology. | |
Molecular Weight |
220.3 g/mol | |
Details | Computed by PubChem 2.1 (PubChem release 2021.05.07) | |
Source | PubChem | |
URL | https://pubchem.ncbi.nlm.nih.gov | |
Description | Data deposited in or computed by PubChem | |
CAS No. |
33527-23-0 | |
Record name | 2,6-Dithia-s-indacene-4,8-dione | |
Source | ChemIDplus | |
URL | https://pubchem.ncbi.nlm.nih.gov/substance/?source=chemidplus&sourceid=0033527230 | |
Description | ChemIDplus is a free, web search system that provides access to the structure and nomenclature authority files used for the identification of chemical substances cited in National Library of Medicine (NLM) databases, including the TOXNET system. | |
Record name | NSC149710 | |
Source | DTP/NCI | |
URL | https://dtp.cancer.gov/dtpstandard/servlet/dwindex?searchtype=NSC&outputformat=html&searchlist=149710 | |
Description | The NCI Development Therapeutics Program (DTP) provides services and resources to the academic and private-sector research communities worldwide to facilitate the discovery and development of new cancer therapeutic agents. | |
Explanation | Unless otherwise indicated, all text within NCI products is free of copyright and may be reused without our permission. Credit the National Cancer Institute as the source. | |
Record name | 2,6-Dithia-s-indacene-4,8-dione | |
Source | EPA DSSTox | |
URL | https://comptox.epa.gov/dashboard/DTXSID90187172 | |
Description | DSSTox provides a high quality public chemistry resource for supporting improved predictive toxicology. | |
Record name | 2,6-DITHIA-S-INDACENE-4,8-DIONE | |
Source | FDA Global Substance Registration System (GSRS) | |
URL | https://gsrs.ncats.nih.gov/ginas/app/beta/substances/XX20GAW81R | |
Description | The FDA Global Substance Registration System (GSRS) enables the efficient and accurate exchange of information on what substances are in regulated products. Instead of relying on names, which vary across regulatory domains, countries, and regions, the GSRS knowledge base makes it possible for substances to be defined by standardized, scientific descriptions. | |
Explanation | Unless otherwise noted, the contents of the FDA website (www.fda.gov), both text and graphics, are not copyrighted. They are in the public domain and may be republished, reprinted and otherwise used freely by anyone without the need to obtain permission from FDA. Credit to the U.S. Food and Drug Administration as the source is appreciated but not required. | |
Synthesis routes and methods
Procedure details
Retrosynthesis Analysis
AI-Powered Synthesis Planning: Our tool employs the Template_relevance Pistachio, Template_relevance Bkms_metabolic, Template_relevance Pistachio_ringbreaker, Template_relevance Reaxys, Template_relevance Reaxys_biocatalysis model, leveraging a vast database of chemical reactions to predict feasible synthetic routes.
One-Step Synthesis Focus: Specifically designed for one-step synthesis, it provides concise and direct routes for your target compounds, streamlining the synthesis process.
Accurate Predictions: Utilizing the extensive PISTACHIO, BKMS_METABOLIC, PISTACHIO_RINGBREAKER, REAXYS, REAXYS_BIOCATALYSIS database, our tool offers high-accuracy predictions, reflecting the latest in chemical research and data.
Strategy Settings
Precursor scoring | Relevance Heuristic |
---|---|
Min. plausibility | 0.01 |
Model | Template_relevance |
Template Set | Pistachio/Bkms_metabolic/Pistachio_ringbreaker/Reaxys/Reaxys_biocatalysis |
Top-N result to add to graph | 6 |
Feasible Synthetic Routes
Disclaimer and Information on In-Vitro Research Products
Please be aware that all articles and product information presented on BenchChem are intended solely for informational purposes. The products available for purchase on BenchChem are specifically designed for in-vitro studies, which are conducted outside of living organisms. In-vitro studies, derived from the Latin term "in glass," involve experiments performed in controlled laboratory settings using cells or tissues. It is important to note that these products are not categorized as medicines or drugs, and they have not received approval from the FDA for the prevention, treatment, or cure of any medical condition, ailment, or disease. We must emphasize that any form of bodily introduction of these products into humans or animals is strictly prohibited by law. It is essential to adhere to these guidelines to ensure compliance with legal and ethical standards in research and experimentation.