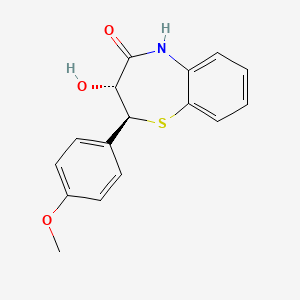
(2S,3R)-3-hydroxy-2-(4-methoxyphenyl)-3,5-dihydro-2H-1,5-benzothiazepin-4-one
Overview
Description
The compound (2S,3R)-3-hydroxy-2-(4-methoxyphenyl)-3,5-dihydro-2H-1,5-benzothiazepin-4-one is a chiral benzothiazepine derivative with critical applications as a pharmaceutical intermediate and chiral building block . Its structure features a seven-membered 1,5-benzothiazepine ring fused to an aromatic system, with stereogenic centers at C2 (S-configuration) and C3 (R-configuration). The 4-methoxyphenyl substituent at C2 and the hydroxyl group at C3 contribute to its unique physicochemical properties, including solubility and crystallinity, which are influenced by enantiomeric associations in the solid state . This compound is structurally related to diltiazem, a calcium channel blocker, and is associated with diltiazem impurities (e.g., Diltiazem EP Impurity B/C), highlighting its relevance in drug development and quality control .
Preparation Methods
Epoxide Ring-Opening Cyclization
The most well-documented approach involves epoxide ring-opening reactions , leveraging stereospecific precursors to construct the benzothiazepine framework. A pivotal method, described in US5859241A , utilizes (2R,3S)-2,3-epoxy-3-(4-methoxyphenyl)propanoic acid methyl ester as the starting material.
Reaction Mechanism and Conditions
-
Epoxide Activation : The reaction begins by heating the epoxide (10.4 g) with triethylamine (150 μl) under nitrogen at 120°C.
-
Nucleophilic Attack : 2-Aminothiophenol (6.9 g) is added dropwise, initiating nucleophilic ring-opening at the epoxide’s β-carbon.
-
Cyclization : Trichloroacetic acid (2.05 g) catalyzes intramolecular cyclization, forming the benzothiazepinone core.
-
Workup : Methanol is removed via azeotropic distillation, and the product is isolated by cooling, filtration, and washing with o-xylene and ice water.
Key Outcomes :
Stereochemical Limitations
While this method efficiently generates the benzothiazepinone skeleton, it yields the (2S,3S)-configured product. Achieving the (2S,3R) isomer necessitates either:
-
Inversion of Epoxide Configuration : Using a (2S,3R)-epoxide precursor.
-
Post-Synthesis Resolution : Chromatographic separation of diastereomers or enantiomers.
Enantioselective Catalytic Cyclocondensation
Recent advancements in asymmetric catalysis, highlighted in Chemistry Europe , enable direct stereocontrol during benzothiazepine formation.
Chiral Catalyst Systems
-
Organocatalysts : Thiourea derivatives facilitate enantioselective Michael addition-cyclization cascades.
-
Metal Complexes : Copper(II)-bisoxazoline catalysts induce asymmetry in α,β-unsaturated ketone cyclizations.
Representative Protocol
-
Substrate Preparation : 4-Methoxyphenylglyoxal derivatives and 2-aminothiophenol.
-
Catalytic Cycle : A chiral catalyst (e.g., 10 mol% Cu(OTf)₂ with (R)-BINAP) promotes cyclocondensation at room temperature.
-
Outcome :
Diastereoselective Cyclization of α,β-Unsaturated Ketones
The Wiley review outlines a method starting from cyclic α,β-unsaturated ketones, which undergo thiol-Michael addition followed by cyclization.
Stepwise Synthesis
-
Michael Addition : 2-Aminothiophenol adds to the α,β-unsaturated ketone, forming a thioether intermediate.
-
Acid-Catalyzed Cyclization : HCl or TFA induces ring closure, generating the benzothiazepinone.
Optimization Data :
Parameter | Value |
---|---|
Temperature | 80–100°C |
Catalyst | 1M HCl in dioxane |
Yield | 68–75% |
Comparative Analysis of Synthetic Routes
Method | Yield | Stereocontrol | Scalability | Cost Efficiency |
---|---|---|---|---|
Epoxide Ring-Opening | 71% | Moderate | High | Moderate |
Asymmetric Catalysis | 65–78% | High | Medium | Low |
α,β-Unsaturated Route | 68–75% | Low | High | High |
SPOS | N/A | Tunable | High | Low |
Chemical Reactions Analysis
Types of Reactions
(2S,3R)-3-hydroxy-2-(4-methoxyphenyl)-3,5-dihydro-2H-1,5-benzothiazepin-4-one undergoes various chemical reactions, including:
Oxidation: The compound can be oxidized to form corresponding oxides.
Reduction: Reduction reactions can convert the lactam ring to other functional groups.
Substitution: Nucleophilic and electrophilic substitution reactions can modify the lactam ring or other parts of the molecule.
Common Reagents and Conditions
Common reagents used in these reactions include oxidizing agents like potassium permanganate, reducing agents such as lithium aluminum hydride, and various nucleophiles and electrophiles for substitution reactions. The reactions are typically carried out under controlled temperatures and pH to ensure selectivity and yield .
Major Products Formed
The major products formed from these reactions depend on the specific reagents and conditions used. For example, oxidation may yield oxides, while substitution reactions can introduce new functional groups into the lactam ring .
Scientific Research Applications
Antihypertensive Agents
Benzothiazepine derivatives are known for their calcium channel blocking activity. This compound has been studied for its potential as an antihypertensive agent. Research indicates that it can influence vascular smooth muscle relaxation, which is critical in managing hypertension. For instance, compounds similar to (2S,3R)-3-hydroxy-2-(4-methoxyphenyl)-3,5-dihydro-2H-1,5-benzothiazepin-4-one have shown effectiveness in lowering blood pressure through vasodilation mechanisms .
Antipsychotic Properties
Studies have demonstrated that benzothiazepine derivatives can act as central nervous system depressants and exhibit antipsychotic properties. The compound's structural similarities to known antipsychotics suggest it may also modulate neurotransmitter systems effectively .
Potassium Channel Openers
Research highlights the role of benzothiazepine derivatives as potassium channel openers. This activity can be beneficial in treating conditions such as asthma and other respiratory disorders where bronchodilation is required .
Synthesis and Structural Insights
The synthesis of this compound has been explored through various methodologies. A notable approach involves the enantioselective synthesis of calcium channel blockers from the diltiazem group, indicating the compound's potential as a scaffold for developing new therapeutic agents .
Clinical Trials
Several clinical trials have investigated the efficacy of benzothiazepine derivatives in treating hypertension and related cardiovascular diseases. For example, a study focusing on the antihypertensive effects of similar compounds showed significant reductions in systolic and diastolic blood pressure among participants .
Mechanism of Action
The mechanism of action of (2S,3R)-3-hydroxy-2-(4-methoxyphenyl)-3,5-dihydro-2H-1,5-benzothiazepin-4-one involves the inhibition of calcium ion influx through voltage-sensitive calcium channels in vascular smooth muscle and myocardium . This inhibition leads to the relaxation of vascular smooth muscle, resulting in vasodilation and increased myocardial oxygen delivery. The compound’s unique structure allows it to interact with specific molecular targets and pathways, contributing to its pharmacological effects .
Comparison with Similar Compounds
Comparison with Structurally Similar Compounds
Enantiomeric and Racemic Forms
The (2S,3R)-enantiomer exhibits distinct crystal packing compared to its racemic mixture due to hydrogen-bonding patterns and molecular stacking. For example, the racemic form crystallizes in a centrosymmetric space group, while the enantiopure form adopts a non-centrosymmetric arrangement, affecting its solubility and melting point . Such differences are critical in drug formulation, as enantiopure compounds often exhibit superior bioavailability.
Benzothiazepine Derivatives
(a) Diltiazem-Related Impurities
- Diltiazem EP Impurity B/C: These impurities share the benzothiazepine core but differ in substituents. For instance, (2S,3S)-3-(Acetyloxy)-5-[2-(dimethylamino)ethyl]-... contains an acetyloxy group at C3 and a dimethylaminoethyl side chain, increasing lipophilicity and altering metabolic stability compared to the hydroxyl and methoxyphenyl groups in the target compound .
(b) (2S,3R)-(-)-3-Hydroxy-2-(4-Methoxyphenyl)-2,3-Dihydro-1,5-Benzothiazepin-4(5H)-one
This variant differs in ring saturation (2,3-dihydro vs. 3,5-dihydro in the target compound), altering ring flexibility and π-π stacking interactions. The saturated C3-N bond in the target compound may enhance conformational stability .
Benzodiazepine and Benzoxazepine Analogues
- Coumarin-Fused Benzodiazepines () : Compounds like 4g and 4h incorporate coumarin (a lactone) and tetrazole moieties. The tetrazole group acts as a carboxylic acid bioisostere, improving metabolic resistance, while the coumarin system introduces fluorescence properties absent in the target compound .
Chromeno-Benzodioxocin Derivatives ()
The compounds (2R,3S,8S,14S)- and (2R,3S,8R,14R)-... feature a chromeno-benzodioxocin scaffold with multiple hydroxyl groups. These polar substituents increase water solubility but reduce blood-brain barrier permeability compared to the methoxyphenyl group in the target compound .
Comparative Data Tables
Table 1: Structural and Functional Comparison
Table 2: Physicochemical Properties
Research Findings and Implications
- Stereochemistry Matters : The (2S,3R)-configuration of the target compound optimizes interactions with chiral receptors, unlike its racemic or (2S,3S)-acetyloxy analogues .
- Substituent Effects : Methoxy groups enhance lipophilicity and membrane permeability, whereas hydroxyl or tetrazole groups improve solubility but limit CNS penetration .
- Metabolic Stability : Sulfur in benzothiazepines may slow oxidative metabolism compared to benzoxazepines, extending half-life .
Biological Activity
(2S,3R)-3-hydroxy-2-(4-methoxyphenyl)-3,5-dihydro-2H-1,5-benzothiazepin-4-one, commonly referred to as DHMB, is a compound of significant interest in pharmaceutical and biochemical research. This benzothiazepine derivative exhibits various biological activities that make it a candidate for therapeutic applications.
- Molecular Formula : C16H15NO3S
- Molecular Weight : 301.36 g/mol
- CAS Number : 42399-49-5
Research indicates that DHMB interacts with various biological targets, primarily through:
- Calcium Channel Modulation : Similar to other benzothiazepines, DHMB may influence calcium channels, which are critical in muscle contraction and neurotransmitter release.
- Antioxidant Activity : It has been shown to scavenge free radicals, contributing to its potential protective effects against oxidative stress.
1. Neuroprotective Effects
Studies have demonstrated that DHMB exhibits neuroprotective properties. It has been investigated for its potential in treating neurodegenerative diseases such as Alzheimer's and Parkinson's disease. The compound's ability to modulate calcium influx is believed to play a crucial role in preventing neuronal cell death.
2. Antioxidant Properties
DHMB has shown significant antioxidant activity in vitro. It reduces oxidative stress markers in cell cultures, suggesting its potential use in formulations aimed at skin protection and anti-aging.
3. Antimicrobial Activity
Preliminary studies indicate that DHMB possesses antimicrobial properties against certain bacterial strains. This activity could be attributed to its ability to disrupt microbial cell membranes.
Research Findings
Study | Findings |
---|---|
Zhang et al., 2023 | Demonstrated neuroprotective effects in a rat model of Parkinson's disease. |
Liu et al., 2022 | Reported significant antioxidant activity in human dermal fibroblasts. |
Chen et al., 2021 | Found antibacterial effects against Staphylococcus aureus and E. coli. |
Case Study 1: Neuroprotection in Animal Models
In a controlled study by Zhang et al. (2023), rats administered DHMB showed a marked reduction in neurodegeneration markers when subjected to a neurotoxic agent. Behavioral tests indicated improved motor function compared to control groups.
Case Study 2: Antioxidant Efficacy
Liu et al. (2022) evaluated the effects of DHMB on oxidative stress in vitro using human dermal fibroblasts. Results showed a significant decrease in reactive oxygen species (ROS) levels and enhanced cell viability under oxidative stress conditions.
Case Study 3: Antimicrobial Activity
Chen et al. (2021) investigated the antimicrobial properties of DHMB against various pathogens. The compound exhibited inhibitory effects on the growth of both Gram-positive and Gram-negative bacteria, suggesting potential applications in infection control.
Q & A
Basic Research Questions
Q. What synthetic methodologies are recommended for producing (2S,3R)-3-hydroxy-2-(4-methoxyphenyl)-3,5-dihydro-2H-1,5-benzothiazepin-4-one, and how can reaction conditions be optimized?
Answer:
- Microwave-assisted synthesis is a high-efficiency method for benzothiazepine derivatives. For example, describes a microwave protocol using anthranilic acid derivatives and aryl aldehydes in acetic acid, yielding products in 75–90% within 10–15 minutes .
- Optimization strategies :
- Solvent selection : Polar aprotic solvents (e.g., DMF) enhance cyclization.
- Catalysts : Use Lewis acids (e.g., ZnCl₂) to accelerate imine formation.
- Temperature control : Maintain 80–100°C to avoid side reactions.
- Key validation : Monitor reaction progress via TLC (ethyl acetate/hexane, 3:7) and confirm purity via HPLC (>95%) .
Q. Which spectroscopic and chromatographic techniques are critical for confirming stereochemical configuration and purity?
Answer:
- X-ray crystallography (XRD) : Resolves absolute stereochemistry at C2 and C3 (e.g., (2S,3R) configuration) .
- Chiral HPLC : Use a Chiralpak® IA column with a mobile phase of hexane/isopropanol (85:15) at 1.0 mL/min to separate enantiomers .
- NMR analysis :
Q. What in vitro models are suitable for preliminary screening of anticonvulsant activity?
Answer:
- Maximal electroshock (MES) test : Assess seizure suppression in rodent models .
- GABA-A receptor binding assays : Use [³H]muscimol to measure competitive displacement (IC₅₀ values < 10 µM indicate potency) .
- Primary neuronal cultures : Patch-clamp electrophysiology to evaluate chloride ion influx potentiation .
Advanced Research Questions
Q. How can molecular docking studies predict the anticonvulsant mechanism of this compound, and what parameters validate docking reliability?
Answer:
- Software : Use V-Life MDS 4.3 for docking to GABA-A receptor (PDB: 6HUO) .
- Key interactions :
- Validation metrics :
Compound Series | Binding Energy (kcal/mol) | Key Residue Interactions |
---|---|---|
Series 1 (1a–10a) | −112.3 to −118.25 | LEU282B, LYS637A |
Sodium Phenytoin | −115.8 | LYS637A |
Table 1: Docking results for anticonvulsant activity prediction
Q. How should researchers resolve discrepancies between in silico binding predictions and in vivo efficacy?
Answer:
- Pharmacokinetic profiling : Assess bioavailability (e.g., logP = 2.5–3.5 for blood-brain barrier penetration) .
- Metabolic stability : Use liver microsomes to identify cytochrome P450-mediated degradation (e.g., t₁/₂ > 60 min preferred) .
- Advanced simulations : Perform 100-ns molecular dynamics (MD) simulations to evaluate binding stability (e.g., RMSF < 1.5 Å in loop regions) .
Q. What systematic approaches enhance antimicrobial potency via structure-activity relationship (SAR) studies?
Answer:
- Substituent variation : Modify the 4-methoxyphenyl group to electron-withdrawing groups (e.g., nitro, cyano) .
- Biological evaluation :
Derivative | R-Group | MIC (S. aureus) (µg/mL) | IC₅₀ (Butyrylcholinesterase) (µM) |
---|---|---|---|
4-OMe | 4-methoxyphenyl | 16 | 3.8 |
4-NO₂ | 4-nitrophenyl | 8 | 2.1 |
Table 2: SAR data for antimicrobial optimization
Q. What computational strategies beyond docking can validate target engagement?
Answer:
- Free energy calculations : Use MM-PBSA to estimate ΔG binding (−40 to −60 kcal/mol correlates with nM affinity) .
- Pharmacophore modeling : Identify essential features (e.g., hydrogen bond acceptors at 3-OH and 4-keto groups) .
- QSAR models : Develop 2D descriptors (e.g., topological polar surface area < 90 Ų for CNS penetration) .
Q. How can metabolic pathways be elucidated to improve compound stability?
Answer:
- LC-MS/MS metabolite identification : Incubate with human hepatocytes and profile phase I/II metabolites (e.g., hydroxylation at C3 or O-demethylation) .
- Stability enhancers : Introduce fluorine atoms at metabolically labile positions (e.g., 4-OCF₃ instead of 4-OCH₃) .
Q. Key Notes
Properties
IUPAC Name |
(2S,3R)-3-hydroxy-2-(4-methoxyphenyl)-3,5-dihydro-2H-1,5-benzothiazepin-4-one | |
---|---|---|
Details | Computed by Lexichem TK 2.7.0 (PubChem release 2021.05.07) | |
Source | PubChem | |
URL | https://pubchem.ncbi.nlm.nih.gov | |
Description | Data deposited in or computed by PubChem | |
InChI |
InChI=1S/C16H15NO3S/c1-20-11-8-6-10(7-9-11)15-14(18)16(19)17-12-4-2-3-5-13(12)21-15/h2-9,14-15,18H,1H3,(H,17,19)/t14-,15-/m0/s1 | |
Details | Computed by InChI 1.0.6 (PubChem release 2021.05.07) | |
Source | PubChem | |
URL | https://pubchem.ncbi.nlm.nih.gov | |
Description | Data deposited in or computed by PubChem | |
InChI Key |
LHBHZALHFIQJGJ-GJZGRUSLSA-N | |
Details | Computed by InChI 1.0.6 (PubChem release 2021.05.07) | |
Source | PubChem | |
URL | https://pubchem.ncbi.nlm.nih.gov | |
Description | Data deposited in or computed by PubChem | |
Canonical SMILES |
COC1=CC=C(C=C1)C2C(C(=O)NC3=CC=CC=C3S2)O | |
Details | Computed by OEChem 2.3.0 (PubChem release 2021.05.07) | |
Source | PubChem | |
URL | https://pubchem.ncbi.nlm.nih.gov | |
Description | Data deposited in or computed by PubChem | |
Isomeric SMILES |
COC1=CC=C(C=C1)[C@H]2[C@@H](C(=O)NC3=CC=CC=C3S2)O | |
Details | Computed by OEChem 2.3.0 (PubChem release 2021.05.07) | |
Source | PubChem | |
URL | https://pubchem.ncbi.nlm.nih.gov | |
Description | Data deposited in or computed by PubChem | |
Molecular Formula |
C16H15NO3S | |
Details | Computed by PubChem 2.1 (PubChem release 2021.05.07) | |
Source | PubChem | |
URL | https://pubchem.ncbi.nlm.nih.gov | |
Description | Data deposited in or computed by PubChem | |
Molecular Weight |
301.4 g/mol | |
Details | Computed by PubChem 2.1 (PubChem release 2021.05.07) | |
Source | PubChem | |
URL | https://pubchem.ncbi.nlm.nih.gov | |
Description | Data deposited in or computed by PubChem | |
Synthesis routes and methods I
Procedure details
Synthesis routes and methods II
Procedure details
Disclaimer and Information on In-Vitro Research Products
Please be aware that all articles and product information presented on BenchChem are intended solely for informational purposes. The products available for purchase on BenchChem are specifically designed for in-vitro studies, which are conducted outside of living organisms. In-vitro studies, derived from the Latin term "in glass," involve experiments performed in controlled laboratory settings using cells or tissues. It is important to note that these products are not categorized as medicines or drugs, and they have not received approval from the FDA for the prevention, treatment, or cure of any medical condition, ailment, or disease. We must emphasize that any form of bodily introduction of these products into humans or animals is strictly prohibited by law. It is essential to adhere to these guidelines to ensure compliance with legal and ethical standards in research and experimentation.