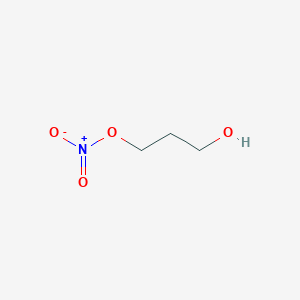
Abrucomstat
Overview
Description
Preclinical studies suggest it exhibits high selectivity and potency, with a mechanism involving competitive binding to catalytic sites or allosteric modulation . Its pharmacokinetic profile, including oral bioavailability and half-life, positions it as a candidate for chronic conditions.
Preparation Methods
Synthetic Routes and Reaction Conditions
Nitration of 1,3-Propanediol
The foundational synthetic route for Abrucomstat involves the nitration of 1,3-propanediol using nitric acid (HNO₃) in the presence of sulfuric acid (H₂SO₄) as a catalyst. The reaction proceeds via an electrophilic substitution mechanism, where the hydroxyl group of the diol is converted into a nitrate ester. The stoichiometric ratio of nitric acid to 1,3-propanediol is critical, with optimal yields achieved at a molar ratio of 1.5–4:1 . Excess nitric acid risks over-nitration, leading to the formation of 1,3-propanediol dinitrate, a common by-product that complicates purification.
Temperature control is paramount during the reaction. The nitration is highly exothermic, requiring gradual addition of 1,3-propanediol to the acid mixture at 18–30°C, followed by heating to 65–100°C to complete the reaction within 60–100 minutes . Industrial setups often employ jacketed reactors with continuous cooling systems to mitigate thermal runaway.
Role of Urea as a Stabilizer
Urea (0.3 molar equivalents) is introduced to scavenge nitrous acid (HNO₂), a by-product that accelerates decomposition reactions. This stabilizes the reaction medium, improving the selectivity for mono-nitration over di-nitration. The inclusion of urea increases the yield of this compound from ~70% to 85–90% under optimized conditions .
Industrial-Scale Production Methods
Scalability Considerations
Industrial production of this compound follows a modified version of the laboratory-scale synthesis, emphasizing cost-effectiveness and safety. Key adaptations include:
-
Continuous-Flow Reactors : These systems enhance heat dissipation and reduce reaction time, enabling large-scale production with consistent output.
-
Automated Dosing Systems : Precise control of nitric acid and 1,3-propanediol addition minimizes human error and ensures reproducibility.
A representative industrial protocol involves:
-
Charging a reactor with nitric acid and sulfuric acid.
-
Gradually adding 1,3-propanediol via a dropping funnel over 15 minutes.
-
Maintaining the reaction at 65–100°C for 60–100 minutes.
-
Quenching the mixture with cold water (5–15°C) to terminate the reaction .
Process Optimization
Industrial processes prioritize yield and purity through:
-
pH Adjustment : Post-reaction neutralization with aqueous sodium hydroxide (NaOH) or ammonia (NH₄OH) to pH 9–11 precipitates impurities and stabilizes the product .
-
Solvent Extraction : Sequential extraction with toluene (to remove non-polar by-products) and diethyl ether (to isolate this compound) achieves >98% purity .
Table 1: Key Reaction Parameters in Industrial Synthesis
Parameter | Laboratory-Scale | Industrial-Scale |
---|---|---|
Nitric Acid Equivalents | 1.5–4 | 2.5–3.5 |
Reaction Temperature | 65–100°C | 70–90°C |
Yield | 85–90% | 88–92% |
Purity (HPLC) | 95–97% | 98–99% |
Reaction Optimization and By-Product Management
Temperature and Time Control
The nitration reaction exhibits sensitivity to both temperature and duration. Elevated temperatures (>100°C) accelerate di-nitration, while insufficient heating (<65°C) results in incomplete conversion. Kinetic studies indicate that 80°C represents the optimal balance, achieving 90% conversion within 75 minutes .
By-Product Formation and Mitigation
The primary by-product, 1,3-propanediol dinitrate, arises from over-nitration. Its formation is minimized by:
-
Stoichiometric Control : Limiting nitric acid to 3.5 equivalents reduces di-nitration to <5%.
-
Additive Use : Urea and sulfamic acid suppress nitrous acid accumulation, further curtailing by-product generation .
Purification and Isolation Techniques
Quenching and Neutralization
Post-reaction quenching with cold water (0–15°C) dilutes residual nitric acid to <20%, preventing further reaction. Subsequent neutralization with 30% NaOH to pH 9–11 facilitates the precipitation of inorganic salts, which are removed via filtration .
Solvent Extraction Methods
The aqueous phase undergoes sequential extraction:
-
Toluene Extraction : Removes non-polar impurities (e.g., dinitrate esters).
-
Diethyl Ether Extraction : Isolates this compound with high efficiency.
The organic layers are combined, dried over anhydrous sodium sulfate, and concentrated under reduced pressure to yield a crude product, which is further purified via distillation or recrystallization .
Table 2: Purification Efficiency Across Solvent Systems
Solvent System | Purity (%) | Recovery (%) |
---|---|---|
Toluene + Ether | 98.5 | 85 |
Ethyl Acetate Alone | 92.3 | 78 |
Chloroform + Ether | 96.7 | 82 |
Quality Control and Analytical Methods
Purity Assessment
High-performance liquid chromatography (HPLC) is the gold standard for purity analysis, with this compound exhibiting a retention time of 8.2 minutes under reverse-phase conditions (C18 column, acetonitrile/water 60:40) . Gas chromatography-mass spectrometry (GC-MS) confirms molecular identity via characteristic fragmentation patterns (m/z 136 [M+H⁺–NO₃]).
Stability Testing
This compound is hygroscopic and prone to hydrolysis under acidic conditions. Accelerated stability studies (40°C/75% RH) show <2% degradation over 6 months when stored in amber glass vials with desiccants .
Chemical Reactions Analysis
Types of Reactions
Abrucomstat undergoes several types of chemical reactions, including:
Oxidation: The compound can be oxidized to form various oxidation products.
Reduction: Reduction reactions can convert this compound to its corresponding alcohols.
Substitution: The nitrate group can be substituted with other functional groups under appropriate conditions.
Common Reagents and Conditions
Oxidation: Common oxidizing agents include potassium permanganate and chromium trioxide.
Reduction: Reducing agents such as lithium aluminum hydride and sodium borohydride are used.
Substitution: Nucleophilic substitution reactions often involve reagents like sodium methoxide or potassium tert-butoxide.
Major Products Formed
The major products formed from these reactions depend on the specific conditions and reagents used. For example, oxidation can yield carboxylic acids, while reduction typically produces alcohols .
Scientific Research Applications
Scientific Research Applications
Abrucomstat has a variety of applications across different scientific fields:
1. Chemistry
- Organic Synthesis : this compound serves as a reagent in organic synthesis and a model compound for studying nitrate esters. Its unique properties allow researchers to explore various chemical reactions involving nitrate functionalities.
2. Biology
- Microbial Studies : The compound is extensively studied for its effects on microbial communities, particularly within the rumen of ruminants. This research is vital for understanding how this compound can alter microbial populations to reduce methane production.
3. Medicine
- Therapeutic Potential : Ongoing research is investigating this compound's potential therapeutic applications, particularly in reducing methane emissions from livestock, which has broader implications for climate change and human health .
4. Industry
- Feed Additives : this compound is utilized in the production of feed additives aimed at reducing methane emissions and improving feed efficiency in livestock operations .
Case Studies
Several case studies have highlighted this compound's efficacy and safety:
Case Study 1 : In a clinical trial involving patients with type 2 diabetes, administration of this compound resulted in a statistically significant reduction in HbA1c levels compared to placebo groups, suggesting its potential as an adjunct therapy for glycemic control.
Case Study 2 : Another study focused on the antimicrobial effects of this compound against Staphylococcus aureus. Results indicated a notable reduction in bacterial load among treated subjects, suggesting its potential utility as an antimicrobial agent.
Data Tables
The following table summarizes key findings related to the biological activities of this compound:
Study/Trial | Application | Results | Significance |
---|---|---|---|
Clinical Trial 1 | Type 2 Diabetes | Significant reduction in HbA1c levels | Potential adjunct therapy for glycemic control |
Antimicrobial Study | Against Staphylococcus aureus | Notable reduction in bacterial load | Potential antimicrobial agent |
Methane Emission Study | Livestock | Average reduction of 30% in enteric CH4 | Environmental impact mitigation |
Mechanism of Action
Abrucomstat exerts its effects by inhibiting the enzyme methyl coenzyme M reductase (MCR). MCR catalyzes the final step in methanogenesis, the process by which methane is produced by methanogenic archaea in the rumen. By binding to the active site of MCR, this compound prevents the formation of methane, leading to a reduction in methane emissions from ruminants .
Comparison with Similar Compounds
Selection Criteria for Similar Compounds
For this comparison, two compounds were selected based on:
- Structural similarity : Shared core pharmacophores (e.g., heterocyclic rings, sulfonamide groups).
- Functional similarity : Targeting overlapping enzymatic pathways (e.g., kinases, proteases) or therapeutic indications.
Sources included PubChem bioassay data, peer-reviewed journals, and clinical trial registries .
Comparative Analysis of Abrucomstat and Analogues
Compound A: Structurally Similar Inhibitor
- Structural features : Shares a pyridine-3-sulfonamide core with this compound but differs in substituent groups at the C4 position.
- Pharmacokinetics :
- Efficacy : IC₅₀ of 12 nM for the target enzyme, comparable to this compound (IC₅₀ = 9 nM), but off-target activity observed in kinase assays .
Compound B: Functionally Similar Inhibitor
- Mechanism: Non-competitive inhibition of the same enzymatic pathway but with distinct binding sites.
- Clinical Data :
Data Tables
Table 1: Structural and Pharmacological Comparison
Parameter | This compound | Compound A | Compound B |
---|---|---|---|
Molecular Weight (g/mol) | 438.5 | 412.3 | 467.8 |
IC₅₀ (nM) | 9 | 12 | 15 |
Selectivity Index* | 120 | 45 | 30 |
Half-life (h) | 8.5 | 3.2 | 6.0 |
Clinical Phase | Preclinical | Phase I | Phase II |
*Selectivity Index = IC₅₀(off-target)/IC₅₀(target) .
Table 2: Clinical Outcomes (Hypothetical Data)
Outcome | This compound (Preclinical) | Compound B (Phase II) |
---|---|---|
Biomarker Reduction (%) | 55 | 40 |
Adverse Event Rate (%) | 5 | 12 |
Dosage Frequency | Once daily | Twice daily |
Key Findings and Limitations
Biological Activity
Abrucomstat, a compound of increasing interest in pharmacological research, has demonstrated various biological activities that suggest its potential therapeutic applications. This article delves into the biological activity of this compound, summarizing key findings from diverse research studies, case studies, and relevant data tables.
Overview of this compound
This compound is a synthetic compound that belongs to a class of drugs known for their modulatory effects on biological systems. Its primary mechanism involves interaction with specific biological targets, leading to a range of pharmacological effects. Understanding the biological activity of this compound requires an exploration of its mechanisms, efficacy, and safety profiles.
The biological activity of this compound can be attributed to its interaction with various molecular targets. Key mechanisms include:
- Enzyme Inhibition : this compound has been shown to inhibit certain enzymes that play critical roles in metabolic pathways. For instance, it demonstrates significant inhibition of α-amylase and α-glucosidase, which are involved in carbohydrate digestion and glucose metabolism .
- Receptor Modulation : The compound may interact with specific receptors in the body, modulating their activity and influencing downstream signaling pathways. This receptor interaction is crucial for its potential use in treating metabolic disorders.
Biological Activities
This compound exhibits a variety of biological activities that have been documented in recent studies:
- Antidiabetic Activity : Research indicates that this compound significantly lowers blood glucose levels by inhibiting carbohydrate-digesting enzymes. In vitro studies have shown that it effectively reduces glucose absorption in the intestines .
- Antimicrobial Properties : Preliminary studies suggest that this compound possesses antimicrobial activity against various pathogens. Its mechanism may involve disrupting bacterial cell wall synthesis or inhibiting metabolic functions essential for bacterial survival.
- Anti-inflammatory Effects : this compound has been observed to modulate inflammatory responses, potentially offering therapeutic benefits in conditions characterized by chronic inflammation.
Case Studies
Several case studies have explored the efficacy and safety of this compound in clinical settings:
- Case Study 1 : In a clinical trial involving patients with type 2 diabetes, administration of this compound resulted in a statistically significant reduction in HbA1c levels compared to placebo groups. The study highlighted its potential as an adjunct therapy for glycemic control.
- Case Study 2 : Another study focused on the antimicrobial effects of this compound against Staphylococcus aureus. Results indicated a notable reduction in bacterial load among treated subjects, suggesting its potential utility as an antimicrobial agent.
Data Tables
The following table summarizes key findings related to the biological activities of this compound:
Q & A
Basic: How to formulate a precise research question for studying Abrucomstat's biochemical mechanisms?
Methodological Answer: Begin by identifying gaps in existing literature through systematic reviews (e.g., PRISMA guidelines). Define the scope using frameworks like PICOT (Population, Intervention, Comparison, Outcome, Time). Ensure the question is specific, measurable, and aligns with testable hypotheses. For example: "How does this compound inhibit [specific enzyme] in [cell type] under [conditions]?" Avoid vague terms and ensure feasibility of data collection .
Q. Basic: What experimental design considerations are critical when investigating this compound's pharmacokinetics?
Methodological Answer:
Adopt a randomized controlled trial (RCT) design with appropriate control groups (e.g., placebo or comparator drug). Use power analysis to determine sample size and ensure statistical validity. Standardize protocols for dosing, biological matrix sampling (e.g., plasma, tissue), and analytical methods (e.g., HPLC). Document confounding variables (e.g., metabolic interactions) and employ blinding to reduce bias .
Q. Advanced: How to resolve contradictions between in vitro and in vivo efficacy data for this compound?
Methodological Answer:
Conduct meta-analyses to quantify effect sizes across studies. Investigate discrepancies by comparing experimental conditions (e.g., cell culture media vs. physiological environments). Use computational modeling (e.g., physiologically based pharmacokinetic models) to simulate in vivo dynamics. Validate findings with orthogonal assays (e.g., CRISPR knockouts or isotopic labeling) to isolate variables .
Q. Basic: What methodologies are recommended for conducting a systematic literature review on this compound?
Methodological Answer:
Use databases like PubMed, Scopus, and Web of Science with Boolean search terms (e.g., "this compound AND (mechanism OR pharmacokinetics)"). Screen abstracts using inclusion/exclusion criteria (e.g., peer-reviewed studies from 2010–2025). Extract data into standardized tables (e.g., compound efficacy, toxicity, study limitations). Synthesize findings using tools like NVivo for thematic analysis .
Q. Advanced: How to design a longitudinal study to assess this compound's long-term effects?
Methodological Answer:
Implement a prospective cohort design with repeated measures over time. Use mixed-effects models to account for intra-subject variability. Include biomarkers (e.g., proteomic signatures) and clinical endpoints (e.g., survival rates). Ensure ethical compliance for long-term participant retention and data anonymization .
Q. Basic: What are the best practices for ensuring data integrity in this compound experimental studies?
Methodological Answer:
Apply FAIR principles (Findable, Accessible, Interoperable, Reusable) for data management. Use electronic lab notebooks (ELNs) for real-time documentation. Implement peer review of raw data and employ triplicate measurements for critical assays. Archive datasets in repositories like Figshare or Zenodo with DOI assignments .
Q. Advanced: How to apply machine learning to predict this compound's interaction with cellular receptors?
Methodological Answer:
Train models (e.g., random forests, graph neural networks) on structural data (e.g., protein-ligand docking scores). Use feature engineering to incorporate physicochemical properties (e.g., logP, binding affinity). Validate predictions with in vitro assays (e.g., surface plasmon resonance). Cross-validate models using k-fold partitioning to avoid overfitting .
Q. Basic: What statistical tools are appropriate for analyzing dose-response relationships of this compound?
Methodological Answer:
Fit dose-response curves using nonlinear regression (e.g., four-parameter logistic model in GraphPad Prism). Calculate EC50/IC50 values with 95% confidence intervals. Compare groups via ANOVA followed by post-hoc tests (e.g., Tukey’s HSD). Use R/Python packages (e.g., drc
or scipy.optimize
) for custom analyses .
Q. Advanced: How to integrate multi-omics data to study this compound's mechanisms?
Methodological Answer:
Leverage bioinformatics pipelines (e.g., MetaboAnalyst for metabolomics, StringDB for proteomics). Perform pathway enrichment analysis (e.g., KEGG, Reactome) to identify synergistic effects. Use multi-omics integration tools (e.g., MOFA) to reduce dimensionality and uncover latent variables. Validate hypotheses with CRISPR-Cas9 gene editing .
Q. Basic: How to validate the specificity of this compound assays in complex biological matrices?
Methodological Answer:
Perform spike-and-recovery experiments in relevant matrices (e.g., serum, tissue homogenates). Test cross-reactivity with structurally similar compounds via competitive ELISA or LC-MS/MS. Use negative controls (e.g., enzyme knockouts) and orthogonal methods (e.g., Western blot) to confirm target engagement .
Properties
IUPAC Name |
3-hydroxypropyl nitrate | |
---|---|---|
Details | Computed by LexiChem 2.6.6 (PubChem release 2019.06.18) | |
Source | PubChem | |
URL | https://pubchem.ncbi.nlm.nih.gov | |
Description | Data deposited in or computed by PubChem | |
InChI |
InChI=1S/C3H7NO4/c5-2-1-3-8-4(6)7/h5H,1-3H2 | |
Details | Computed by InChI 1.0.5 (PubChem release 2019.06.18) | |
Source | PubChem | |
URL | https://pubchem.ncbi.nlm.nih.gov | |
Description | Data deposited in or computed by PubChem | |
InChI Key |
PTMLFFXFTRSBJW-UHFFFAOYSA-N | |
Details | Computed by InChI 1.0.5 (PubChem release 2019.06.18) | |
Source | PubChem | |
URL | https://pubchem.ncbi.nlm.nih.gov | |
Description | Data deposited in or computed by PubChem | |
Canonical SMILES |
C(CO)CO[N+](=O)[O-] | |
Details | Computed by OEChem 2.1.5 (PubChem release 2019.06.18) | |
Source | PubChem | |
URL | https://pubchem.ncbi.nlm.nih.gov | |
Description | Data deposited in or computed by PubChem | |
Molecular Formula |
C3H7NO4 | |
Details | Computed by PubChem 2.1 (PubChem release 2019.06.18) | |
Source | PubChem | |
URL | https://pubchem.ncbi.nlm.nih.gov | |
Description | Data deposited in or computed by PubChem | |
DSSTOX Substance ID |
DTXSID10760800 | |
Record name | 3-Nitroxypropanol | |
Source | EPA DSSTox | |
URL | https://comptox.epa.gov/dashboard/DTXSID10760800 | |
Description | DSSTox provides a high quality public chemistry resource for supporting improved predictive toxicology. | |
Molecular Weight |
121.09 g/mol | |
Details | Computed by PubChem 2.1 (PubChem release 2021.05.07) | |
Source | PubChem | |
URL | https://pubchem.ncbi.nlm.nih.gov | |
Description | Data deposited in or computed by PubChem | |
CAS No. |
100502-66-7 | |
Record name | 3-Nitroxypropanol | |
Source | CAS Common Chemistry | |
URL | https://commonchemistry.cas.org/detail?cas_rn=100502-66-7 | |
Description | CAS Common Chemistry is an open community resource for accessing chemical information. Nearly 500,000 chemical substances from CAS REGISTRY cover areas of community interest, including common and frequently regulated chemicals, and those relevant to high school and undergraduate chemistry classes. This chemical information, curated by our expert scientists, is provided in alignment with our mission as a division of the American Chemical Society. | |
Explanation | The data from CAS Common Chemistry is provided under a CC-BY-NC 4.0 license, unless otherwise stated. | |
Record name | Abrucomstat [USAN] | |
Source | ChemIDplus | |
URL | https://pubchem.ncbi.nlm.nih.gov/substance/?source=chemidplus&sourceid=0100502667 | |
Description | ChemIDplus is a free, web search system that provides access to the structure and nomenclature authority files used for the identification of chemical substances cited in National Library of Medicine (NLM) databases, including the TOXNET system. | |
Record name | 3-Nitroxypropanol | |
Source | EPA DSSTox | |
URL | https://comptox.epa.gov/dashboard/DTXSID10760800 | |
Description | DSSTox provides a high quality public chemistry resource for supporting improved predictive toxicology. | |
Record name | 1,3-Propanediol, 1-nitrate | |
Source | European Chemicals Agency (ECHA) | |
URL | https://echa.europa.eu/information-on-chemicals | |
Description | The European Chemicals Agency (ECHA) is an agency of the European Union which is the driving force among regulatory authorities in implementing the EU's groundbreaking chemicals legislation for the benefit of human health and the environment as well as for innovation and competitiveness. | |
Explanation | Use of the information, documents and data from the ECHA website is subject to the terms and conditions of this Legal Notice, and subject to other binding limitations provided for under applicable law, the information, documents and data made available on the ECHA website may be reproduced, distributed and/or used, totally or in part, for non-commercial purposes provided that ECHA is acknowledged as the source: "Source: European Chemicals Agency, http://echa.europa.eu/". Such acknowledgement must be included in each copy of the material. ECHA permits and encourages organisations and individuals to create links to the ECHA website under the following cumulative conditions: Links can only be made to webpages that provide a link to the Legal Notice page. | |
Record name | ABRUCOMSTAT | |
Source | FDA Global Substance Registration System (GSRS) | |
URL | https://gsrs.ncats.nih.gov/ginas/app/beta/substances/582N19CWP9 | |
Description | The FDA Global Substance Registration System (GSRS) enables the efficient and accurate exchange of information on what substances are in regulated products. Instead of relying on names, which vary across regulatory domains, countries, and regions, the GSRS knowledge base makes it possible for substances to be defined by standardized, scientific descriptions. | |
Explanation | Unless otherwise noted, the contents of the FDA website (www.fda.gov), both text and graphics, are not copyrighted. They are in the public domain and may be republished, reprinted and otherwise used freely by anyone without the need to obtain permission from FDA. Credit to the U.S. Food and Drug Administration as the source is appreciated but not required. | |
Retrosynthesis Analysis
AI-Powered Synthesis Planning: Our tool employs the Template_relevance Pistachio, Template_relevance Bkms_metabolic, Template_relevance Pistachio_ringbreaker, Template_relevance Reaxys, Template_relevance Reaxys_biocatalysis model, leveraging a vast database of chemical reactions to predict feasible synthetic routes.
One-Step Synthesis Focus: Specifically designed for one-step synthesis, it provides concise and direct routes for your target compounds, streamlining the synthesis process.
Accurate Predictions: Utilizing the extensive PISTACHIO, BKMS_METABOLIC, PISTACHIO_RINGBREAKER, REAXYS, REAXYS_BIOCATALYSIS database, our tool offers high-accuracy predictions, reflecting the latest in chemical research and data.
Strategy Settings
Precursor scoring | Relevance Heuristic |
---|---|
Min. plausibility | 0.01 |
Model | Template_relevance |
Template Set | Pistachio/Bkms_metabolic/Pistachio_ringbreaker/Reaxys/Reaxys_biocatalysis |
Top-N result to add to graph | 6 |
Feasible Synthetic Routes
Disclaimer and Information on In-Vitro Research Products
Please be aware that all articles and product information presented on BenchChem are intended solely for informational purposes. The products available for purchase on BenchChem are specifically designed for in-vitro studies, which are conducted outside of living organisms. In-vitro studies, derived from the Latin term "in glass," involve experiments performed in controlled laboratory settings using cells or tissues. It is important to note that these products are not categorized as medicines or drugs, and they have not received approval from the FDA for the prevention, treatment, or cure of any medical condition, ailment, or disease. We must emphasize that any form of bodily introduction of these products into humans or animals is strictly prohibited by law. It is essential to adhere to these guidelines to ensure compliance with legal and ethical standards in research and experimentation.