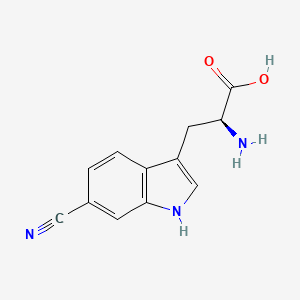
6-Cyanotryptophan
Overview
Description
6-Cyanotryptophan is a derivative of the amino acid tryptophan, characterized by the presence of a nitrile group (-CN) at the sixth position of the indole ring. This modification imparts unique spectroscopic properties to the compound, making it a valuable tool in various scientific research applications, particularly in the fields of chemistry, biology, and medicine.
Preparation Methods
Synthetic Routes and Reaction Conditions: One common method is the use of a palladium-catalyzed cyanation reaction, where a suitable precursor, such as 6-bromo-tryptophan, is treated with a cyanide source under specific conditions . The reaction conditions often include the use of a palladium catalyst, a base, and a solvent like dimethylformamide (DMF) or dimethyl sulfoxide (DMSO).
Industrial Production Methods: Industrial production of 6-Cyanotryptophan may involve similar synthetic routes but on a larger scale. The process is optimized for higher yields and purity, often employing continuous flow reactors and advanced purification techniques such as high-performance liquid chromatography (HPLC).
Chemical Reactions Analysis
Types of Reactions: 6-Cyanotryptophan undergoes various chemical reactions, including:
Oxidation: The nitrile group can be oxidized to form amides or carboxylic acids under specific conditions.
Reduction: The nitrile group can be reduced to primary amines using reducing agents like lithium aluminum hydride (LiAlH4).
Substitution: The indole ring can undergo electrophilic substitution reactions, allowing for further functionalization.
Common Reagents and Conditions:
Oxidation: Reagents such as potassium permanganate (KMnO4) or hydrogen peroxide (H2O2) in acidic or basic conditions.
Reduction: Reducing agents like LiAlH4 or catalytic hydrogenation using palladium on carbon (Pd/C).
Substitution: Electrophiles like halogens or nitro groups in the presence of Lewis acids.
Major Products:
Oxidation: Amides or carboxylic acids.
Reduction: Primary amines.
Substitution: Various substituted indoles depending on the electrophile used.
Scientific Research Applications
6-Cyanotryptophan is widely used in scientific research due to its unique spectroscopic properties. Some key applications include:
Mechanism of Action
The mechanism by which 6-Cyanotryptophan exerts its effects is primarily through its spectroscopic properties. The nitrile group acts as a non-invasive probe that can provide information on local electrostatics and hydrogen bonding environments. This allows researchers to study the structural dynamics of proteins and other biomolecules in real-time .
Comparison with Similar Compounds
6-Cyanotryptophan is unique among tryptophan derivatives due to its dual infrared and fluorescence spectroscopic properties. Similar compounds include:
4-Cyanotryptophan: Another nitrile-containing tryptophan derivative with similar spectroscopic properties but different positional substitution.
5-Cyanotryptophan: Similar to this compound but with the nitrile group at the fifth position, affecting its spectroscopic behavior.
These compounds are valuable tools in studying protein dynamics and interactions, but this compound’s specific substitution pattern provides unique insights into local electrostatics and hydrogen bonding environments.
Properties
IUPAC Name |
(2S)-2-amino-3-(6-cyano-1H-indol-3-yl)propanoic acid | |
---|---|---|
Details | Computed by Lexichem TK 2.7.0 (PubChem release 2021.05.07) | |
Source | PubChem | |
URL | https://pubchem.ncbi.nlm.nih.gov | |
Description | Data deposited in or computed by PubChem | |
InChI |
InChI=1S/C12H11N3O2/c13-5-7-1-2-9-8(4-10(14)12(16)17)6-15-11(9)3-7/h1-3,6,10,15H,4,14H2,(H,16,17)/t10-/m0/s1 | |
Details | Computed by InChI 1.0.6 (PubChem release 2021.05.07) | |
Source | PubChem | |
URL | https://pubchem.ncbi.nlm.nih.gov | |
Description | Data deposited in or computed by PubChem | |
InChI Key |
QFVPXIGESRCWHK-JTQLQIEISA-N | |
Details | Computed by InChI 1.0.6 (PubChem release 2021.05.07) | |
Source | PubChem | |
URL | https://pubchem.ncbi.nlm.nih.gov | |
Description | Data deposited in or computed by PubChem | |
Canonical SMILES |
C1=CC2=C(C=C1C#N)NC=C2CC(C(=O)O)N | |
Details | Computed by OEChem 2.3.0 (PubChem release 2021.05.07) | |
Source | PubChem | |
URL | https://pubchem.ncbi.nlm.nih.gov | |
Description | Data deposited in or computed by PubChem | |
Isomeric SMILES |
C1=CC2=C(C=C1C#N)NC=C2C[C@@H](C(=O)O)N | |
Details | Computed by OEChem 2.3.0 (PubChem release 2021.05.07) | |
Source | PubChem | |
URL | https://pubchem.ncbi.nlm.nih.gov | |
Description | Data deposited in or computed by PubChem | |
Molecular Formula |
C12H11N3O2 | |
Details | Computed by PubChem 2.1 (PubChem release 2021.05.07) | |
Source | PubChem | |
URL | https://pubchem.ncbi.nlm.nih.gov | |
Description | Data deposited in or computed by PubChem | |
Molecular Weight |
229.23 g/mol | |
Details | Computed by PubChem 2.1 (PubChem release 2021.05.07) | |
Source | PubChem | |
URL | https://pubchem.ncbi.nlm.nih.gov | |
Description | Data deposited in or computed by PubChem | |
Retrosynthesis Analysis
AI-Powered Synthesis Planning: Our tool employs the Template_relevance Pistachio, Template_relevance Bkms_metabolic, Template_relevance Pistachio_ringbreaker, Template_relevance Reaxys, Template_relevance Reaxys_biocatalysis model, leveraging a vast database of chemical reactions to predict feasible synthetic routes.
One-Step Synthesis Focus: Specifically designed for one-step synthesis, it provides concise and direct routes for your target compounds, streamlining the synthesis process.
Accurate Predictions: Utilizing the extensive PISTACHIO, BKMS_METABOLIC, PISTACHIO_RINGBREAKER, REAXYS, REAXYS_BIOCATALYSIS database, our tool offers high-accuracy predictions, reflecting the latest in chemical research and data.
Strategy Settings
Precursor scoring | Relevance Heuristic |
---|---|
Min. plausibility | 0.01 |
Model | Template_relevance |
Template Set | Pistachio/Bkms_metabolic/Pistachio_ringbreaker/Reaxys/Reaxys_biocatalysis |
Top-N result to add to graph | 6 |
Feasible Synthetic Routes
Disclaimer and Information on In-Vitro Research Products
Please be aware that all articles and product information presented on BenchChem are intended solely for informational purposes. The products available for purchase on BenchChem are specifically designed for in-vitro studies, which are conducted outside of living organisms. In-vitro studies, derived from the Latin term "in glass," involve experiments performed in controlled laboratory settings using cells or tissues. It is important to note that these products are not categorized as medicines or drugs, and they have not received approval from the FDA for the prevention, treatment, or cure of any medical condition, ailment, or disease. We must emphasize that any form of bodily introduction of these products into humans or animals is strictly prohibited by law. It is essential to adhere to these guidelines to ensure compliance with legal and ethical standards in research and experimentation.