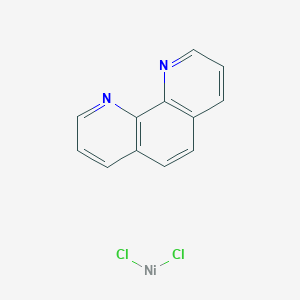
Ni(phen)cl2
Overview
Description
Nickel(II) chloride with 1,10-phenanthroline, commonly referred to as Ni(phen)Cl2, is a coordination compound where nickel(II) ions are coordinated with 1,10-phenanthroline ligands and chloride ions. This compound is known for its distinctive structural and chemical properties, making it a subject of interest in various fields of scientific research.
Scientific Research Applications
Ni(phen)Cl2 has a wide range of applications in scientific research:
Preparation Methods
Synthetic Routes and Reaction Conditions
Ni(phen)Cl2 can be synthesized through the reaction of nickel(II) chloride with 1,10-phenanthroline in an appropriate solvent. One common method involves dissolving nickel(II) chloride in methanol and adding 1,10-phenanthroline to the solution. The mixture is then stirred and heated to facilitate the formation of the coordination complex. The resulting product is typically isolated by filtration and recrystallization .
Industrial Production Methods
While specific industrial production methods for this compound are not extensively documented, the general approach involves large-scale synthesis using similar reaction conditions as in laboratory settings. The process may be optimized for higher yields and purity, often involving automated systems for mixing, heating, and crystallization.
Chemical Reactions Analysis
Types of Reactions
Ni(phen)Cl2 undergoes various chemical reactions, including:
Substitution Reactions: The chloride ions in this compound can be substituted by other ligands, such as nitrate or acetate, under appropriate conditions.
Oxidation-Reduction Reactions: Nickel(II) in this compound can participate in redox reactions, where it can be reduced to nickel(I) or oxidized to nickel(III) depending on the reagents used.
Common Reagents and Conditions
Substitution Reactions: Common reagents include silver nitrate (for nitrate substitution) and sodium acetate (for acetate substitution).
Oxidation-Reduction Reactions: Reducing agents like sodium borohydride can reduce nickel(II) to nickel(I), while oxidizing agents like hydrogen peroxide can oxidize it to nickel(III).
Major Products
Substitution Reactions: Products include nickel(II) complexes with different ligands, such as Ni(phen)(NO3)2 or Ni(phen)(OAc)2.
Oxidation-Reduction Reactions: Products include nickel(I) or nickel(III) complexes, depending on the specific redox conditions.
Mechanism of Action
The mechanism by which Ni(phen)Cl2 exerts its effects involves the coordination of nickel(II) ions with 1,10-phenanthroline ligands. This coordination alters the electronic properties of the nickel center, making it more reactive in various chemical processes. The phenanthroline ligands also stabilize the nickel center, allowing it to participate in redox reactions and catalysis .
Comparison with Similar Compounds
Similar Compounds
Nickel(II) chloride with 2,2’-bipyridine (Ni(bipy)Cl2): Similar to Ni(phen)Cl2, but with 2,2’-bipyridine as the ligand.
Nickel(II) chloride with ethylenediamine (Ni(en)Cl2): Uses ethylenediamine as the ligand, resulting in different coordination properties.
Uniqueness
This compound is unique due to the specific electronic and steric properties imparted by the 1,10-phenanthroline ligands. These properties make it particularly effective in catalysis and redox reactions compared to other nickel(II) complexes .
Properties
IUPAC Name |
dichloronickel;1,10-phenanthroline | |
---|---|---|
Details | Computed by LexiChem 2.6.6 (PubChem release 2019.06.18) | |
Source | PubChem | |
URL | https://pubchem.ncbi.nlm.nih.gov | |
Description | Data deposited in or computed by PubChem | |
InChI |
InChI=1S/C12H8N2.2ClH.Ni/c1-3-9-5-6-10-4-2-8-14-12(10)11(9)13-7-1;;;/h1-8H;2*1H;/q;;;+2/p-2 | |
Details | Computed by InChI 1.0.5 (PubChem release 2019.06.18) | |
Source | PubChem | |
URL | https://pubchem.ncbi.nlm.nih.gov | |
Description | Data deposited in or computed by PubChem | |
InChI Key |
RMTGLTCLJRSHSB-UHFFFAOYSA-L | |
Details | Computed by InChI 1.0.5 (PubChem release 2019.06.18) | |
Source | PubChem | |
URL | https://pubchem.ncbi.nlm.nih.gov | |
Description | Data deposited in or computed by PubChem | |
Canonical SMILES |
C1=CC2=C(C3=C(C=CC=N3)C=C2)N=C1.Cl[Ni]Cl | |
Details | Computed by OEChem 2.1.5 (PubChem release 2019.06.18) | |
Source | PubChem | |
URL | https://pubchem.ncbi.nlm.nih.gov | |
Description | Data deposited in or computed by PubChem | |
Molecular Formula |
C12H8Cl2N2Ni | |
Details | Computed by PubChem 2.1 (PubChem release 2019.06.18) | |
Source | PubChem | |
URL | https://pubchem.ncbi.nlm.nih.gov | |
Description | Data deposited in or computed by PubChem | |
Molecular Weight |
309.80 g/mol | |
Details | Computed by PubChem 2.1 (PubChem release 2021.05.07) | |
Source | PubChem | |
URL | https://pubchem.ncbi.nlm.nih.gov | |
Description | Data deposited in or computed by PubChem | |
Retrosynthesis Analysis
AI-Powered Synthesis Planning: Our tool employs the Template_relevance Pistachio, Template_relevance Bkms_metabolic, Template_relevance Pistachio_ringbreaker, Template_relevance Reaxys, Template_relevance Reaxys_biocatalysis model, leveraging a vast database of chemical reactions to predict feasible synthetic routes.
One-Step Synthesis Focus: Specifically designed for one-step synthesis, it provides concise and direct routes for your target compounds, streamlining the synthesis process.
Accurate Predictions: Utilizing the extensive PISTACHIO, BKMS_METABOLIC, PISTACHIO_RINGBREAKER, REAXYS, REAXYS_BIOCATALYSIS database, our tool offers high-accuracy predictions, reflecting the latest in chemical research and data.
Strategy Settings
Precursor scoring | Relevance Heuristic |
---|---|
Min. plausibility | 0.01 |
Model | Template_relevance |
Template Set | Pistachio/Bkms_metabolic/Pistachio_ringbreaker/Reaxys/Reaxys_biocatalysis |
Top-N result to add to graph | 6 |
Feasible Synthetic Routes
Disclaimer and Information on In-Vitro Research Products
Please be aware that all articles and product information presented on BenchChem are intended solely for informational purposes. The products available for purchase on BenchChem are specifically designed for in-vitro studies, which are conducted outside of living organisms. In-vitro studies, derived from the Latin term "in glass," involve experiments performed in controlled laboratory settings using cells or tissues. It is important to note that these products are not categorized as medicines or drugs, and they have not received approval from the FDA for the prevention, treatment, or cure of any medical condition, ailment, or disease. We must emphasize that any form of bodily introduction of these products into humans or animals is strictly prohibited by law. It is essential to adhere to these guidelines to ensure compliance with legal and ethical standards in research and experimentation.