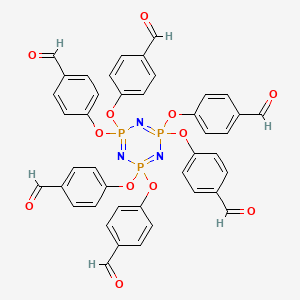
Cyclotriphosphazene-pmmh-6 dendrimer generation 0.5
- Click on QUICK INQUIRY to receive a quote from our team of experts.
- With the quality product at a COMPETITIVE price, you can focus more on your research.
Overview
Description
Cyclotriphosphazene-pmmh-6 dendrimer generation 0.5 is a type of dendrimer, which is a synthetic, tree-like macromolecule. Dendrimers are characterized by their highly branched, three-dimensional structure, which provides a high degree of surface functionality and versatility. This compound specifically features a cyclotriphosphazene core with phenoxymethyl (methylhydrazono) groups attached to it. This compound is known for its stability, high solubility in various solvents, and significant dipole moments .
Preparation Methods
The synthesis of cyclotriphosphazene-pmmh-6 dendrimer generation 0.5 involves multiple steps. The process typically begins with the preparation of the cyclotriphosphazene core, followed by the attachment of phenoxymethyl (methylhydrazono) groups. The reaction conditions often include the use of solvents such as ethanol and specific catalysts to facilitate the reactions. Industrial production methods may involve scaling up these reactions while ensuring the purity and consistency of the final product .
Chemical Reactions Analysis
Cyclotriphosphazene-pmmh-6 dendrimer generation 0.5 can undergo various chemical reactions, including oxidation, reduction, and substitution reactions. Common reagents used in these reactions include oxidizing agents like hydrogen peroxide, reducing agents such as sodium borohydride, and substitution reagents like alkyl halides. The major products formed from these reactions depend on the specific conditions and reagents used, but they often involve modifications to the phenoxymethyl (methylhydrazono) groups or the cyclotriphosphazene core .
Scientific Research Applications
Cyclotriphosphazene-pmmh-6 dendrimer generation 0.5 has a wide range of scientific research applications. In chemistry, it is used as a building block for the synthesis of more complex dendrimers and polymers. In biology and medicine, it has potential applications in drug delivery systems due to its ability to encapsulate therapeutic agents and release them in a controlled manner. Additionally, its high surface functionality makes it useful in the development of sensors and diagnostic tools. In industry, it can be used in the production of advanced materials with specific properties, such as high thermal stability and conductivity .
Mechanism of Action
The mechanism of action of cyclotriphosphazene-pmmh-6 dendrimer generation 0.5 involves its interaction with molecular targets through its surface functional groups. These interactions can lead to various effects, such as the encapsulation of molecules, catalysis of reactions, or binding to specific receptors. The pathways involved depend on the specific application and the nature of the interactions between the dendrimer and its targets .
Comparison with Similar Compounds
Cyclotriphosphazene-pmmh-6 dendrimer generation 0.5 can be compared with other similar compounds, such as cyclotriphosphazene-pmmh-6 dendrimer generation 1.0 and other phosphorous dendrimers with cyclotriphosphazene cores. The uniqueness of this compound lies in its specific surface functional groups and its generation level, which influence its properties and applications. Similar compounds include cyclotriphosphazene-phenoxymethyl (methylhydrazono) dendrimer generation 1.0, poly(bis(4-carboxyphenoxy)phosphazene), and poly(bis(phenoxy)phosphazene) .
Properties
IUPAC Name |
4-[[2,4,4,6,6-pentakis(4-formylphenoxy)-1,3,5-triaza-2λ5,4λ5,6λ5-triphosphacyclohexa-1,3,5-trien-2-yl]oxy]benzaldehyde |
Source
|
---|---|---|
Details | Computed by Lexichem TK 2.7.0 (PubChem release 2021.05.07) | |
Source | PubChem | |
URL | https://pubchem.ncbi.nlm.nih.gov | |
Description | Data deposited in or computed by PubChem | |
InChI |
InChI=1S/C42H30N3O12P3/c46-25-31-1-13-37(14-2-31)52-58(53-38-15-3-32(26-47)4-16-38)43-59(54-39-17-5-33(27-48)6-18-39,55-40-19-7-34(28-49)8-20-40)45-60(44-58,56-41-21-9-35(29-50)10-22-41)57-42-23-11-36(30-51)12-24-42/h1-30H |
Source
|
Details | Computed by InChI 1.0.6 (PubChem release 2021.05.07) | |
Source | PubChem | |
URL | https://pubchem.ncbi.nlm.nih.gov | |
Description | Data deposited in or computed by PubChem | |
InChI Key |
IUQFLKMWSWBJHE-UHFFFAOYSA-N |
Source
|
Details | Computed by InChI 1.0.6 (PubChem release 2021.05.07) | |
Source | PubChem | |
URL | https://pubchem.ncbi.nlm.nih.gov | |
Description | Data deposited in or computed by PubChem | |
Canonical SMILES |
C1=CC(=CC=C1C=O)OP2(=NP(=NP(=N2)(OC3=CC=C(C=C3)C=O)OC4=CC=C(C=C4)C=O)(OC5=CC=C(C=C5)C=O)OC6=CC=C(C=C6)C=O)OC7=CC=C(C=C7)C=O |
Source
|
Details | Computed by OEChem 2.3.0 (PubChem release 2021.05.07) | |
Source | PubChem | |
URL | https://pubchem.ncbi.nlm.nih.gov | |
Description | Data deposited in or computed by PubChem | |
Molecular Formula |
C42H30N3O12P3 |
Source
|
Details | Computed by PubChem 2.1 (PubChem release 2021.05.07) | |
Source | PubChem | |
URL | https://pubchem.ncbi.nlm.nih.gov | |
Description | Data deposited in or computed by PubChem | |
Molecular Weight |
861.6 g/mol |
Source
|
Details | Computed by PubChem 2.1 (PubChem release 2021.05.07) | |
Source | PubChem | |
URL | https://pubchem.ncbi.nlm.nih.gov | |
Description | Data deposited in or computed by PubChem | |
Disclaimer and Information on In-Vitro Research Products
Please be aware that all articles and product information presented on BenchChem are intended solely for informational purposes. The products available for purchase on BenchChem are specifically designed for in-vitro studies, which are conducted outside of living organisms. In-vitro studies, derived from the Latin term "in glass," involve experiments performed in controlled laboratory settings using cells or tissues. It is important to note that these products are not categorized as medicines or drugs, and they have not received approval from the FDA for the prevention, treatment, or cure of any medical condition, ailment, or disease. We must emphasize that any form of bodily introduction of these products into humans or animals is strictly prohibited by law. It is essential to adhere to these guidelines to ensure compliance with legal and ethical standards in research and experimentation.