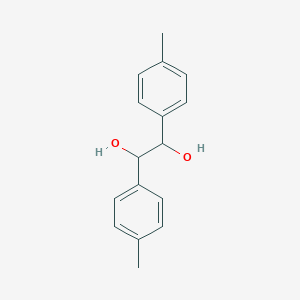
1,2-di-p-tolylethane-1,2-diol
- Click on QUICK INQUIRY to receive a quote from our team of experts.
- With the quality product at a COMPETITIVE price, you can focus more on your research.
Overview
Description
1,2-di-p-tolylethane-1,2-diol: is an organic compound with the molecular formula C16H18O2 It is a diol, meaning it contains two hydroxyl groups (-OH) attached to adjacent carbon atoms The compound is characterized by the presence of two p-tolyl groups (4-methylphenyl) attached to the ethane backbone
Preparation Methods
Synthetic Routes and Reaction Conditions: 1,2-di-p-tolylethane-1,2-diol can be synthesized through several methods. One common method involves the reduction of 1,2-di-p-tolylethane-1,2-dione using sodium borohydride (NaBH4) in an alcohol solvent such as ethanol. The reaction typically proceeds at room temperature and yields the desired diol product.
Industrial Production Methods: Industrial production of this compound may involve similar reduction processes but on a larger scale. The use of catalytic hydrogenation with palladium on carbon (Pd/C) as a catalyst in the presence of hydrogen gas (H2) can also be employed for efficient production. The reaction conditions are optimized to ensure high yield and purity of the product.
Chemical Reactions Analysis
Types of Reactions: 1,2-di-p-tolylethane-1,2-diol undergoes various chemical reactions, including:
Oxidation: The diol can be oxidized to form 1,2-di-p-tolylethane-1,2-dione using oxidizing agents such as chromium trioxide (CrO3) or potassium permanganate (KMnO4).
Reduction: The compound can be further reduced to form the corresponding hydrocarbon, 1,2-di-p-tolylethane, using strong reducing agents like lithium aluminum hydride (LiAlH4).
Substitution: The hydroxyl groups can be substituted with other functional groups through reactions with reagents like thionyl chloride (SOCl2) to form the corresponding chlorides.
Common Reagents and Conditions:
Oxidation: Chromium trioxide (CrO3) in acetic acid (CH3COOH) at room temperature.
Reduction: Lithium aluminum hydride (LiAlH4) in dry ether at low temperatures.
Substitution: Thionyl chloride (SOCl2) in the presence of pyridine at room temperature.
Major Products:
Oxidation: 1,2-di-p-tolylethane-1,2-dione.
Reduction: 1,2-di-p-tolylethane.
Substitution: 1,2-di-p-tolylethane-1,2-dichloride.
Scientific Research Applications
1,2-di-p-tolylethane-1,2-diol has several scientific research applications, including:
Chemistry: Used as a building block in organic synthesis for the preparation of more complex molecules.
Biology: Investigated for its potential biological activity and interactions with enzymes and proteins.
Medicine: Explored for its potential therapeutic properties and as a precursor for drug development.
Industry: Utilized in the production of polymers, resins, and other industrial chemicals due to its diol functionality.
Mechanism of Action
The mechanism of action of 1,2-di-p-tolylethane-1,2-diol involves its interaction with various molecular targets and pathways. The hydroxyl groups can form hydrogen bonds with other molecules, influencing their reactivity and stability. In biological systems, the compound may interact with enzymes and proteins, affecting their function and activity. The specific pathways and targets depend on the context of its application and the nature of the interacting molecules.
Comparison with Similar Compounds
1,2-diphenylethane-1,2-diol: Similar structure but with phenyl groups instead of p-tolyl groups.
1,2-di-p-tolylethane-1,2-dione: The oxidized form of 1,2-di-p-tolylethane-1,2-diol.
1,2-bis(4-fluorophenyl)ethane-1,2-diol: Similar structure with fluorine substituents on the phenyl rings.
Uniqueness: this compound is unique due to the presence of p-tolyl groups, which impart specific electronic and steric properties. These properties can influence the compound’s reactivity, solubility, and interactions with other molecules, making it distinct from other similar diols.
Properties
IUPAC Name |
1,2-bis(4-methylphenyl)ethane-1,2-diol |
Source
|
---|---|---|
Details | Computed by LexiChem 2.6.6 (PubChem release 2019.06.18) | |
Source | PubChem | |
URL | https://pubchem.ncbi.nlm.nih.gov | |
Description | Data deposited in or computed by PubChem | |
InChI |
InChI=1S/C16H18O2/c1-11-3-7-13(8-4-11)15(17)16(18)14-9-5-12(2)6-10-14/h3-10,15-18H,1-2H3 |
Source
|
Details | Computed by InChI 1.0.5 (PubChem release 2019.06.18) | |
Source | PubChem | |
URL | https://pubchem.ncbi.nlm.nih.gov | |
Description | Data deposited in or computed by PubChem | |
InChI Key |
ZYLCBIATOIJBEH-UHFFFAOYSA-N |
Source
|
Details | Computed by InChI 1.0.5 (PubChem release 2019.06.18) | |
Source | PubChem | |
URL | https://pubchem.ncbi.nlm.nih.gov | |
Description | Data deposited in or computed by PubChem | |
Canonical SMILES |
CC1=CC=C(C=C1)C(C(C2=CC=C(C=C2)C)O)O |
Source
|
Details | Computed by OEChem 2.1.5 (PubChem release 2019.06.18) | |
Source | PubChem | |
URL | https://pubchem.ncbi.nlm.nih.gov | |
Description | Data deposited in or computed by PubChem | |
Molecular Formula |
C16H18O2 |
Source
|
Details | Computed by PubChem 2.1 (PubChem release 2019.06.18) | |
Source | PubChem | |
URL | https://pubchem.ncbi.nlm.nih.gov | |
Description | Data deposited in or computed by PubChem | |
DSSTOX Substance ID |
DTXSID60449739 |
Source
|
Record name | 1,2-Ethanediol, 1,2-bis(4-methylphenyl)- | |
Source | EPA DSSTox | |
URL | https://comptox.epa.gov/dashboard/DTXSID60449739 | |
Description | DSSTox provides a high quality public chemistry resource for supporting improved predictive toxicology. | |
Molecular Weight |
242.31 g/mol |
Source
|
Details | Computed by PubChem 2.1 (PubChem release 2021.05.07) | |
Source | PubChem | |
URL | https://pubchem.ncbi.nlm.nih.gov | |
Description | Data deposited in or computed by PubChem | |
CAS No. |
24133-59-3 |
Source
|
Record name | 1,2-Ethanediol, 1,2-bis(4-methylphenyl)- | |
Source | EPA DSSTox | |
URL | https://comptox.epa.gov/dashboard/DTXSID60449739 | |
Description | DSSTox provides a high quality public chemistry resource for supporting improved predictive toxicology. | |
Disclaimer and Information on In-Vitro Research Products
Please be aware that all articles and product information presented on BenchChem are intended solely for informational purposes. The products available for purchase on BenchChem are specifically designed for in-vitro studies, which are conducted outside of living organisms. In-vitro studies, derived from the Latin term "in glass," involve experiments performed in controlled laboratory settings using cells or tissues. It is important to note that these products are not categorized as medicines or drugs, and they have not received approval from the FDA for the prevention, treatment, or cure of any medical condition, ailment, or disease. We must emphasize that any form of bodily introduction of these products into humans or animals is strictly prohibited by law. It is essential to adhere to these guidelines to ensure compliance with legal and ethical standards in research and experimentation.