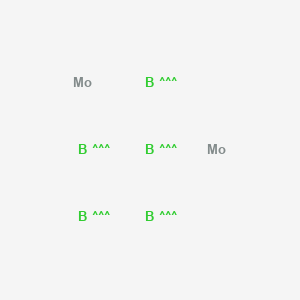
Molybdenum boride (Mo2B5)
Overview
Description
Molybdenum boride (Mo2B5) is an intermetallic compound composed of molybdenum and boron. It belongs to a class of materials known as borides, which are characterized by their high hardness, high melting points, and excellent wear resistance. Molybdenum boride is particularly noted for its unique combination of mechanical and chemical properties, making it a valuable material in various industrial applications.
Preparation Methods
Synthetic Routes and Reaction Conditions: Molybdenum boride can be synthesized through several methods, including:
Self-Propagating High-Temperature Synthesis (SHS): This method uses a thermite-type starting mixture consisting of molybdenum oxide, aluminum, boron oxide, and elemental boron powders.
Chemical Vapor Deposition (CVD): This involves the deposition of boron and boron oxide powders onto molybdenum foils using hydrogen gas as both the carrier and reducing gas.
Industrial Production Methods: Industrial production of molybdenum boride typically involves large-scale boriding processes, where molybdenum powder is reacted with boron sources in high-temperature furnaces. The process parameters, such as temperature, atmosphere, and reaction time, are carefully controlled to ensure the formation of high-purity molybdenum boride.
Chemical Reactions Analysis
Types of Reactions: Molybdenum boride undergoes various chemical reactions, including:
Oxidation: Molybdenum boride can be oxidized at high temperatures to form molybdenum oxide and boron oxide.
Reduction: It can be reduced using hydrogen gas to form molybdenum and boron.
Substitution: Molybdenum boride can participate in substitution reactions with other metal borides or carbides.
Common Reagents and Conditions:
Oxidation: Typically occurs at temperatures above 800°C in the presence of oxygen.
Reduction: Conducted at high temperatures (around 1000°C) using hydrogen gas as the reducing agent.
Substitution: Involves reacting molybdenum boride with other metal powders or carbides at high temperatures.
Major Products Formed:
Oxidation: Molybdenum oxide (MoO3) and boron oxide (B2O3).
Reduction: Elemental molybdenum (Mo) and boron (B).
Substitution: Formation of mixed metal borides or carbides.
Scientific Research Applications
Molybdenum boride has a wide range of scientific research applications, including:
Electrocatalysis: It is used as a catalyst for the hydrogen evolution reaction (HER) due to its excellent electrocatalytic properties.
Wear-Resistant Coatings: Due to its high hardness and wear resistance, molybdenum boride is used in coatings for cutting tools and other high-performance materials.
High-Temperature Ceramics: It is used in the production of ultra-high-temperature ceramics for aerospace and industrial applications.
Mechanism of Action
Molybdenum boride can be compared with other metal borides such as tungsten boride (W2B5), titanium boride (TiB2), and zirconium boride (ZrB2). These compounds share similar properties, including high hardness, high melting points, and excellent wear resistance. molybdenum boride is unique in its combination of mechanical strength and catalytic activity, making it particularly suitable for applications in electrocatalysis and high-temperature environments .
Comparison with Similar Compounds
- Tungsten boride (W2B5)
- Titanium boride (TiB2)
- Zirconium boride (ZrB2)
Molybdenum boride stands out due to its superior electrocatalytic properties and its ability to form stable, high-surface-area structures, which are advantageous for various industrial and scientific applications.
Biological Activity
Molybdenum boride (Mo2B5) is a compound that has garnered attention for its unique properties and potential applications, particularly in catalysis and materials science. This article explores the biological activity of Mo2B5, focusing on its electrocatalytic properties, structural characteristics, and synthesis methods. The findings are supported by data tables, case studies, and relevant research.
Overview of Molybdenum Boride
Molybdenum borides are transition metal borides that exhibit a variety of stoichiometries and crystal structures, including α-MoB, β-MoB, Mo2B5, and others. These compounds are characterized by their hardness, electrical conductivity, and catalytic activity, making them suitable for various industrial applications.
Electrocatalytic Activity
One of the most significant biological activities of Mo2B5 is its role as an electrocatalyst in the hydrogen evolution reaction (HER). Research has shown that molybdenum borides can serve as effective alternatives to noble metals like platinum due to their unique electronic properties and stability under harsh conditions.
Case Study: Hydrogen Evolution Reaction
A study conducted by Park et al. (2017) synthesized several molybdenum borides, including Mo2B5, and evaluated their electrocatalytic performance. The results indicated that while Mo2B5 exhibited lower HER activity compared to other phases like β-MoB and MoB2, it still demonstrated significant catalytic potential in acidic solutions. The study highlighted the boron-dependency of these materials, suggesting that the presence of boron enhances the catalytic properties of molybdenum borides .
Structural Characteristics
The structure of Mo2B5 has been investigated using advanced techniques such as X-ray diffraction (XRD) and scanning transmission electron microscopy (STEM). These studies reveal that Mo2B5 possesses a unique crystal structure that contributes to its mechanical strength and catalytic properties.
Structural Analysis
Research by Tang et al. (2020) focused on the structural determination of boron-rich molybdenum borides. The findings indicated that Mo2B5 exhibits short-range ordering within its crystal lattice, which is crucial for its mechanical properties and reactivity .
Synthesis Methods
The synthesis of molybdenum borides can be achieved through various methods, including:
- Arc Melting : A common method for producing bulk samples.
- Induction Thermal Plasma : This technique allows for the synthesis of nanoparticles with controlled morphology.
- Carbo/Borothermal Reduction : Employed to enhance the purity and crystalline quality of the final product.
Each method has its advantages in terms of scalability and material characteristics. For instance, induction thermal plasma synthesis has been shown to yield high-purity nanoparticles suitable for catalytic applications .
Applications in Biological Contexts
While the primary focus of research on Mo2B5 has been on its physical and chemical properties, there is growing interest in exploring its biological applications. The stability of molybdenum borides under extreme conditions suggests potential use in biosensing technologies and environmental monitoring through techniques like surface-enhanced Raman scattering (SERS).
Surface-Enhanced Raman Scattering (SERS)
Recent studies have demonstrated that molybdenum boride ceramics can serve as effective SERS substrates. For example, β-MoB exhibited a remarkable SERS enhancement factor comparable to gold nanoparticles when tested with Rhodamine 6G probes. This property could be leveraged for detecting biological molecules in complex environments .
Properties
InChI |
InChI=1S/5B.2Mo | |
---|---|---|
Source | PubChem | |
URL | https://pubchem.ncbi.nlm.nih.gov | |
Description | Data deposited in or computed by PubChem | |
InChI Key |
RYKWKNGQFAVABI-UHFFFAOYSA-N | |
Source | PubChem | |
URL | https://pubchem.ncbi.nlm.nih.gov | |
Description | Data deposited in or computed by PubChem | |
Canonical SMILES |
[B].[B].[B].[B].[B].[Mo].[Mo] | |
Source | PubChem | |
URL | https://pubchem.ncbi.nlm.nih.gov | |
Description | Data deposited in or computed by PubChem | |
Molecular Formula |
B5Mo2 | |
Source | PubChem | |
URL | https://pubchem.ncbi.nlm.nih.gov | |
Description | Data deposited in or computed by PubChem | |
Molecular Weight |
246.0 g/mol | |
Source | PubChem | |
URL | https://pubchem.ncbi.nlm.nih.gov | |
Description | Data deposited in or computed by PubChem | |
CAS No. |
12007-97-5 | |
Record name | Molybdenum boride (Mo2B5) | |
Source | ChemIDplus | |
URL | https://pubchem.ncbi.nlm.nih.gov/substance/?source=chemidplus&sourceid=0012007975 | |
Description | ChemIDplus is a free, web search system that provides access to the structure and nomenclature authority files used for the identification of chemical substances cited in National Library of Medicine (NLM) databases, including the TOXNET system. | |
Record name | Molybdenum boride (Mo2B5) | |
Source | EPA Chemicals under the TSCA | |
URL | https://www.epa.gov/chemicals-under-tsca | |
Description | EPA Chemicals under the Toxic Substances Control Act (TSCA) collection contains information on chemicals and their regulations under TSCA, including non-confidential content from the TSCA Chemical Substance Inventory and Chemical Data Reporting. | |
Record name | Dimolybdenum pentaboride | |
Source | European Chemicals Agency (ECHA) | |
URL | https://echa.europa.eu/substance-information/-/substanceinfo/100.031.372 | |
Description | The European Chemicals Agency (ECHA) is an agency of the European Union which is the driving force among regulatory authorities in implementing the EU's groundbreaking chemicals legislation for the benefit of human health and the environment as well as for innovation and competitiveness. | |
Explanation | Use of the information, documents and data from the ECHA website is subject to the terms and conditions of this Legal Notice, and subject to other binding limitations provided for under applicable law, the information, documents and data made available on the ECHA website may be reproduced, distributed and/or used, totally or in part, for non-commercial purposes provided that ECHA is acknowledged as the source: "Source: European Chemicals Agency, http://echa.europa.eu/". Such acknowledgement must be included in each copy of the material. ECHA permits and encourages organisations and individuals to create links to the ECHA website under the following cumulative conditions: Links can only be made to webpages that provide a link to the Legal Notice page. | |
Disclaimer and Information on In-Vitro Research Products
Please be aware that all articles and product information presented on BenchChem are intended solely for informational purposes. The products available for purchase on BenchChem are specifically designed for in-vitro studies, which are conducted outside of living organisms. In-vitro studies, derived from the Latin term "in glass," involve experiments performed in controlled laboratory settings using cells or tissues. It is important to note that these products are not categorized as medicines or drugs, and they have not received approval from the FDA for the prevention, treatment, or cure of any medical condition, ailment, or disease. We must emphasize that any form of bodily introduction of these products into humans or animals is strictly prohibited by law. It is essential to adhere to these guidelines to ensure compliance with legal and ethical standards in research and experimentation.