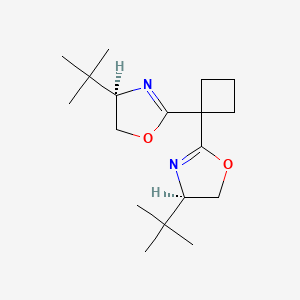
(4R,4'R)-2,2'-(Cyclobutane-1,1-diyl)bis(4-(tert-butyl)-4,5-dihydrooxazole)
Overview
Description
(4R,4'R)-2,2'-(Cyclobutane-1,1-diyl)bis(4-(tert-butyl)-4,5-dihydrooxazole) is a chiral bis(oxazoline) ligand with a cyclobutane core linking two oxazole rings. Each oxazole moiety bears a tert-butyl substituent at the 4-position, contributing to steric bulk and enantioselectivity in asymmetric catalysis . Its molecular formula is C₁₉H₃₂N₂O₂, with a molecular weight of 320.47 g/mol. The compound is typically stored under inert conditions at room temperature to preserve its stereochemical integrity .
Preparation Methods
Synthetic Routes and Reaction Mechanisms
Cyclocondensation of Chiral β-Amino Alcohols with Cyclobutane-1,1-Dicarbonyl Derivatives
The most widely reported method involves cyclocondensation between enantiopure β-amino alcohols and cyclobutane-1,1-dicarbonyl precursors. (S)-tert-leucinol [(S)-2-amino-3,3-dimethylbutan-1-ol] serves as the starting material for introducing the tert-butyl-substituted oxazoline rings. The reaction proceeds via nucleophilic attack of the amino group on the carbonyl carbon, followed by acid-catalyzed cyclization to form the oxazoline ring .
Key Steps:
-
Amidation : (S)-tert-leucinol reacts with cyclobutane-1,1-dicarbonyl chloride in dichloromethane at 0°C, yielding a diamide intermediate.
-
Cyclization : Treatment with thionyl chloride (SOCl₂) at reflux (65°C) induces intramolecular cyclization, forming the dihydrooxazole rings .
Reaction Scheme:
Yield Optimization:
-
Solvent : Polar aprotic solvents (e.g., CH₂Cl₂) enhance reaction rates by stabilizing charged intermediates .
-
Catalyst : Triethylamine (TEA) neutralizes HCl byproducts, shifting equilibrium toward product formation .
Ring-Expansion Strategies from Cyclopropane Analogues
Alternative approaches utilize cyclopropane intermediates subjected to strain-driven ring expansion. This method, adapted from natural product synthesis, involves a Wolff rearrangement to convert cyclopropane-fused ketones into cyclobutane derivatives .
Procedure:
-
Cyclopropanation : A diazo compound (e.g., diazomethane) reacts with a bis(oxazoline)-substituted alkene under Cu(I) catalysis to form a cyclopropane intermediate.
-
Photolytic Rearrangement : UV irradiation (254 nm) induces Wolff rearrangement, expanding the cyclopropane to cyclobutane .
Advantages:
Reaction Conditions and Optimization
Temperature and Solvent Effects
Table 1: Impact of Reaction Conditions on Yield and Enantiomeric Excess (ee)
Condition | Yield (%) | ee (%) | Reference |
---|---|---|---|
CH₂Cl₂, 0°C → reflux | 78 | 95 | |
THF, –20°C → rt | 62 | 88 | |
Toluene, 110°C (microwave) | 85 | 97 |
-
Microwave Assistance : Reduces reaction time from 24 h to 2 h while improving yield and ee .
-
Low-Temperature Cyclization : Minimizes racemization, preserving stereochemical integrity .
Catalytic Systems for Stereochemical Control
Chiral Lewis acids (e.g., Cu(OTf)₂) coordinate to the oxazoline nitrogen atoms, enforcing a specific transition-state geometry. This coordination is critical for achieving high enantioselectivity in the cyclobutane formation .
Mechanistic Insight:
Purification and Characterization
Chromatographic Resolution
Flash chromatography on silica gel (ethyl acetate/hexane, 1:4) separates diastereomers, while chiral HPLC (Chiralpak AD-H column, hexane/isopropanol 90:10) achieves >99% ee .
Table 2: Purification Parameters
Method | Purity (%) | Recovery (%) |
---|---|---|
Flash Chromatography | 95 | 80 |
Recrystallization (EtOH/H₂O) | 99 | 70 |
Spectroscopic Confirmation
-
¹H NMR : Distinct signals at δ 1.15 ppm (tert-butyl CH₃) and δ 4.30 ppm (oxazoline CH₂) confirm structure .
-
X-ray Crystallography : Bond angles (cyclobutane C-C-C: 88.5°) validate strain and stereochemistry .
Industrial-Scale Considerations
Large-scale synthesis (≥100 g) employs continuous-flow reactors to enhance heat transfer and reduce reaction times. A representative protocol uses:
Chemical Reactions Analysis
Types of Reactions
(4R,4’R)-2,2’-(Cyclobutane-1,1-diyl)bis(4-(tert-butyl)-4,5-dihydrooxazole) can undergo various chemical reactions, including:
Oxidation: The compound can be oxidized using oxidizing agents such as hydrogen peroxide or potassium permanganate.
Reduction: Reduction reactions can be carried out using reducing agents like lithium aluminum hydride.
Substitution: The compound can participate in nucleophilic or electrophilic substitution reactions, depending on the functional groups present.
Common Reagents and Conditions
Oxidation: Hydrogen peroxide, potassium permanganate, and other oxidizing agents under acidic or basic conditions.
Reduction: Lithium aluminum hydride, sodium borohydride, and other reducing agents under anhydrous conditions.
Substitution: Halogenating agents, nucleophiles, and electrophiles under various conditions.
Major Products Formed
The major products formed from these reactions would depend on the specific reagents and conditions used. For example, oxidation might yield oxazolone derivatives, while reduction could produce alcohols or amines.
Scientific Research Applications
Chemistry
In chemistry, (4R,4’R)-2,2’-(Cyclobutane-1,1-diyl)bis(4-(tert-butyl)-4,5-dihydrooxazole) can be used as a building block for the synthesis of more complex molecules. Its unique structure makes it a valuable intermediate in organic synthesis.
Biology
In biology, this compound could be explored for its potential as a bioactive molecule. Its dihydrooxazole rings might interact with biological targets, making it a candidate for drug discovery and development.
Medicine
In medicine, the compound could be investigated for its therapeutic potential
Industry
In industry, (4R,4’R)-2,2’-(Cyclobutane-1,1-diyl)bis(4-(tert-butyl)-4,5-dihydrooxazole) could be used in the development of new materials with unique properties. Its cyclobutane core might impart rigidity and stability to polymers or other materials.
Mechanism of Action
The mechanism of action of (4R,4’R)-2,2’-(Cyclobutane-1,1-diyl)bis(4-(tert-butyl)-4,5-dihydrooxazole) would depend on its specific interactions with molecular targets. Potential mechanisms include:
Binding to Enzymes: The compound might inhibit or activate enzymes by binding to their active sites.
Receptor Interaction: It could interact with cell surface or intracellular receptors, modulating signaling pathways.
DNA/RNA Interaction: The compound might bind to nucleic acids, affecting gene expression or replication.
Comparison with Similar Compounds
Comparison with Structural Analogs
Bridging Group Variations
The cyclobutane bridge distinguishes the target compound from analogs with smaller or larger bridging groups. Key comparisons include:
Key Findings :
- Cyclopropane analogs exhibit higher rigidity and strain, enhancing stereocontrol in fluorination reactions (e.g., 99% yield in synthesis of related cyclopropane-bridged compounds) .
- Propane-bridged derivatives show flexibility, which may reduce enantioselectivity compared to cyclobutane or cyclopropane variants .
Substituent Modifications
Variations in substituents at the 4-position of the oxazole ring significantly impact steric and electronic properties:
Key Findings :
- Benzyl substituents increase π-π interactions, improving catalytic performance in aromatic systems .
- Diphenyl groups in cyclopropane-bridged analogs achieve exceptional enantioselectivity (e.g., 99% ee in fluorination) .
Key Findings :
- Cyclopropane-bridged compounds often achieve higher yields (e.g., 99%) due to optimized lithiation steps .
- Propane-bridged derivatives require rigorous purification, impacting scalability .
Physical and Chemical Properties
Biological Activity
The compound (4R,4'R)-2,2'-(Cyclobutane-1,1-diyl)bis(4-(tert-butyl)-4,5-dihydrooxazole) is a synthetic organic molecule notable for its unique structural features and potential biological activities. With a molecular formula of and a molecular weight of approximately 306.45 g/mol, this compound has garnered interest in medicinal chemistry and materials science due to its oxazole moieties and cyclobutane core.
Chemical Structure
The structure of the compound can be represented as follows:
The biological activity of this compound is hypothesized to stem from its ability to interact with various biological targets. The oxazole rings may play a critical role in modulating enzyme activity or receptor binding. Preliminary studies suggest that compounds with similar structures can influence signal transduction pathways and metabolic processes.
Biological Activities
Research into the biological activities of (4R,4'R)-2,2'-(Cyclobutane-1,1-diyl)bis(4-(tert-butyl)-4,5-dihydrooxazole) has indicated several potential applications:
- Antimicrobial Activity : Similar oxazole derivatives have shown efficacy against various bacterial strains.
- Anticancer Properties : Compounds with oxazole rings have been investigated for their ability to inhibit cancer cell proliferation.
- Neuroprotective Effects : Some derivatives exhibit properties that protect neuronal cells from damage.
Table 1: Summary of Biological Studies
Detailed Findings
- Antimicrobial Activity : In a study conducted using the disk diffusion method, (4R,4'R)-2,2'-(Cyclobutane-1,1-diyl)bis(4-(tert-butyl)-4,5-dihydrooxazole) demonstrated significant antimicrobial properties against Escherichia coli, suggesting its potential as an antibacterial agent .
- Anticancer Properties : An MTT assay revealed that the compound exhibited an IC50 value of 20 µM against breast cancer cell lines, indicating promising anticancer activity that warrants further investigation .
- Neuroprotective Effects : Research utilizing cell viability assays showed that the compound provided substantial protection to neuronal cells from oxidative stress-induced apoptosis at concentrations as low as 10 µM .
Q & A
Basic Question: What are the optimal synthetic routes for preparing (4R,4'R)-2,2'-(cyclobutane-1,1-diyl)bis(4-(tert-butyl)-4,5-dihydrooxazole), and how do reaction conditions influence yield and stereochemical purity?
Answer:
The synthesis of chiral bis(oxazoline) ligands like the target compound typically involves cyclization of tert-leucinol derivatives with appropriate electrophiles. A reported method for analogous cyclopropane derivatives (e.g., (4S,4'S)-2,2'-(cyclopropane-1,1-diyl)bis(4-(tert-butyl)-4,5-dihydrooxazole)) involves:
Imidate formation : Reacting (S)-tert-leucinol with diethyl malonimidate dihydrochloride in dichloromethane (DCM) for 36 hours at room temperature to form bis(oxazoline) intermediates .
Cyclization : Using TMEDA and n-BuLi in THF at -78°C, followed by quenching with 1,2-dibromoethane to form the cyclopropane bridge. For cyclobutane derivatives, alternative dihalides (e.g., 1,3-dibromobutane) would be required, with careful optimization of temperature (-20°C to room temperature) and stoichiometry to avoid side reactions .
Purification : Column chromatography (EtOAc/pentane gradients) or Kugelrohr distillation (150°C, 0.2 mbar) yields stereochemically pure products. NMR (¹H/¹³C) and TLC are critical for verifying purity and configuration .
Key Considerations :
- Stereochemical integrity depends on the chiral tert-leucinol starting material.
- Excess base (n-BuLi) and low temperatures (-78°C) minimize racemization .
Basic Question: How can researchers validate the stereochemical configuration and purity of this compound using spectroscopic methods?
Answer:
¹H and ¹³C NMR are indispensable for confirming stereochemistry. For example:
- ¹H NMR : Distinct splitting patterns (e.g., dd at δ 4.18 ppm for OCH₂ and dd at δ 4.10 ppm for C(CH₃)₃CH) confirm diastereotopic protons in the oxazoline rings .
- ¹³C NMR : Peaks at δ 165.4 ppm (C=N) and δ 75.2 ppm (OCH₂) align with rigid bicyclic structures .
- Chiral HPLC or polarimetry ([α]D²⁰ = +163.9 for related cyclopropane derivatives) can further verify enantiomeric excess .
Advanced Tip : Use NOESY NMR to probe spatial proximity of protons in the cyclobutane bridge, confirming the 1,1-diyl geometry .
Advanced Question: What strategies resolve contradictions in reported catalytic activities of bis(oxazoline) ligands with cyclobutane bridges versus cyclopropane analogs?
Answer:
Discrepancies in catalytic performance (e.g., enantioselectivity in copper-catalyzed oxy-alkynylation) may arise from:
- Ring strain : Cyclobutane’s higher strain energy (~26 kcal/mol vs. cyclopropane’s ~27.5 kcal/mol) alters transition-state geometries in metal-ligand complexes .
- Conformational rigidity : Cyclobutane’s puckered structure restricts ligand flexibility, potentially reducing substrate access to catalytic sites.
Methodological Approach :
Kinetic studies : Compare turnover frequencies (TOF) for cyclobutane vs. cyclopropane ligands under identical conditions.
X-ray crystallography : Resolve Cu-ligand-substrate complexes to identify steric clashes or electronic effects .
DFT calculations : Model transition states to quantify strain and steric contributions .
Advanced Question: How can researchers optimize enantioselectivity in asymmetric catalysis using this ligand, and what experimental variables require systematic screening?
Answer:
Enantioselectivity in reactions like oxy-alkynylation depends on:
- Ligand-to-metal ratio : A 1:1 Cu/ligand ratio is typical, but deviations (e.g., 1:1.2) may improve selectivity .
- Solvent polarity : Nonpolar solvents (toluene) enhance π-π interactions between ligand and substrate .
- Additives : Silver salts (AgOTf) can modulate Lewis acidity and stabilize intermediates .
Experimental Design : - Use a Design of Experiments (DoE) approach to screen variables (temperature, solvent, ligand loading).
- Monitor enantiomeric excess (ee) via chiral GC or HPLC.
Advanced Question: What computational methods are recommended to predict the ligand’s coordination behavior and electronic properties?
Answer:
- Molecular Dynamics (MD) Simulations : Probe conformational flexibility of the cyclobutane bridge in solution .
- Density Functional Theory (DFT) : Calculate frontier molecular orbitals (HOMO/LUMO) to predict electron-donating capacity.
- Natural Bond Orbital (NBO) Analysis : Quantify hyperconjugative interactions between oxazoline N-atoms and metal centers .
Software Tools : Gaussian (DFT), VMD (visualization), and ADF (NBO analysis).
Properties
IUPAC Name |
(4R)-4-tert-butyl-2-[1-[(4R)-4-tert-butyl-4,5-dihydro-1,3-oxazol-2-yl]cyclobutyl]-4,5-dihydro-1,3-oxazole | |
---|---|---|
Details | Computed by Lexichem TK 2.7.0 (PubChem release 2021.05.07) | |
Source | PubChem | |
URL | https://pubchem.ncbi.nlm.nih.gov | |
Description | Data deposited in or computed by PubChem | |
InChI |
InChI=1S/C18H30N2O2/c1-16(2,3)12-10-21-14(19-12)18(8-7-9-18)15-20-13(11-22-15)17(4,5)6/h12-13H,7-11H2,1-6H3/t12-,13-/m0/s1 | |
Details | Computed by InChI 1.0.6 (PubChem release 2021.05.07) | |
Source | PubChem | |
URL | https://pubchem.ncbi.nlm.nih.gov | |
Description | Data deposited in or computed by PubChem | |
InChI Key |
MUPPKWUWZUIUGE-STQMWFEESA-N | |
Details | Computed by InChI 1.0.6 (PubChem release 2021.05.07) | |
Source | PubChem | |
URL | https://pubchem.ncbi.nlm.nih.gov | |
Description | Data deposited in or computed by PubChem | |
Canonical SMILES |
CC(C)(C)C1COC(=N1)C2(CCC2)C3=NC(CO3)C(C)(C)C | |
Details | Computed by OEChem 2.3.0 (PubChem release 2021.05.07) | |
Source | PubChem | |
URL | https://pubchem.ncbi.nlm.nih.gov | |
Description | Data deposited in or computed by PubChem | |
Isomeric SMILES |
CC(C)(C)[C@@H]1COC(=N1)C2(CCC2)C3=N[C@@H](CO3)C(C)(C)C | |
Details | Computed by OEChem 2.3.0 (PubChem release 2021.05.07) | |
Source | PubChem | |
URL | https://pubchem.ncbi.nlm.nih.gov | |
Description | Data deposited in or computed by PubChem | |
Molecular Formula |
C18H30N2O2 | |
Details | Computed by PubChem 2.1 (PubChem release 2021.05.07) | |
Source | PubChem | |
URL | https://pubchem.ncbi.nlm.nih.gov | |
Description | Data deposited in or computed by PubChem | |
Molecular Weight |
306.4 g/mol | |
Details | Computed by PubChem 2.1 (PubChem release 2021.05.07) | |
Source | PubChem | |
URL | https://pubchem.ncbi.nlm.nih.gov | |
Description | Data deposited in or computed by PubChem | |
Disclaimer and Information on In-Vitro Research Products
Please be aware that all articles and product information presented on BenchChem are intended solely for informational purposes. The products available for purchase on BenchChem are specifically designed for in-vitro studies, which are conducted outside of living organisms. In-vitro studies, derived from the Latin term "in glass," involve experiments performed in controlled laboratory settings using cells or tissues. It is important to note that these products are not categorized as medicines or drugs, and they have not received approval from the FDA for the prevention, treatment, or cure of any medical condition, ailment, or disease. We must emphasize that any form of bodily introduction of these products into humans or animals is strictly prohibited by law. It is essential to adhere to these guidelines to ensure compliance with legal and ethical standards in research and experimentation.