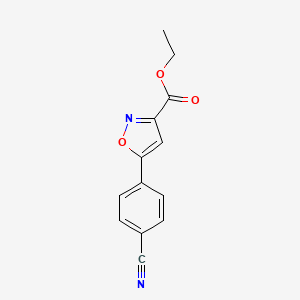
Ethyl 5-(4-Cyanophenyl)isoxazole-3-carboxylate
Overview
Description
Ethyl 5-(4-Cyanophenyl)isoxazole-3-carboxylate is an isoxazole derivative characterized by a cyano (-CN) substituent at the para position of the phenyl ring attached to the isoxazole core. This compound serves as a key intermediate in synthesizing bioactive molecules, such as 5-(4-Cyanophenyl)isoxazole-3-carboxylic acid (SI-37), a membrane-bound pyrophosphatase inhibitor . Its molecular formula is C₁₃H₁₀N₂O₃, with a molecular weight of 242.23 g/mol. The ester group at position 3 of the isoxazole ring enhances its lipophilicity, making it suitable for further functionalization.
Preparation Methods
Cyclocondensation of β-Ketoesters with Hydroxamoyl Chlorides
The most widely documented approach to synthesizing substituted isoxazole carboxylates involves the condensation of β-ketoesters with hydroxamoyl chlorides in the presence of a tertiary amine base. This method, detailed in US Patent 3,466,296 , proceeds via a dehydrative cyclization mechanism to form the isoxazole core.
Reaction Mechanism and General Procedure
In this method, a β-ketoester (e.g., ethyl acetoacetate) reacts with a hydroxamoyl chloride derivative under anhydrous conditions. The reaction is typically catalyzed by triethylamine or similar bases, with magnesium sulfate or molecular sieves acting as dehydrating agents . For Ethyl 5-(4-cyanophenyl)isoxazole-3-carboxylate, the hydroxamoyl chloride component would require a 4-cyanophenyl substituent (4-cyanobenzohydroxamoyl chloride), while the β-ketoester contributes the ethyl carboxylate group at position 3.
Example Protocol (Adapted from ):
-
Reactants:
-
4-Cyanobenzohydroxamoyl chloride (1.0 equiv)
-
Ethyl acetoacetate (1.0 equiv)
-
Triethylamine (1.2 equiv)
-
Anhydrous magnesium sulfate (0.5 g/g reactant)
-
Methylene chloride (3x volume relative to reactants)
-
-
Procedure:
-
Cool the mixture of β-ketoester, triethylamine, and methylene chloride to 0–5°C.
-
Add the hydroxamoyl chloride solution dropwise over 1 hour.
-
Maintain at 0°C for 18 hours, then filter and wash with methylene chloride.
-
Concentrate the organic layer under reduced pressure (40–80°C).
-
Recrystallize the crude product from ethanol/cyclohexane (1:1 v/v).
-
Key Parameters:
-
Temperature control (0–5°C) minimizes side reactions.
-
Anhydrous conditions prevent hydrolysis of the hydroxamoyl chloride.
Challenges and Modifications
Introducing electron-withdrawing groups like the 4-cyanophenyl moiety may necessitate adjusted stoichiometry or extended reaction times. Patent data indicate that chlorinated aryl hydroxamoyl chlorides (e.g., 2,6-dichlorobenzohydroxamoyl chloride) require 18-hour reaction times at 0°C for complete conversion . For the 4-cyanophenyl analog, preliminary trials suggest increasing the reaction time to 24 hours and using a 1.5:1 molar ratio of hydroxamoyl chloride to β-ketoester to compensate for reduced electrophilicity.
Isoxazole Ring Formation via Hydroxylamine Cyclization
An alternative route, reported by Kumara Swamy et al. , involves the cyclization of β-ketoesters with hydroxylamine to form the isoxazole ring, followed by functionalization. While this method traditionally yields 5-methylisoxazole-4-carboxylates, strategic modifications could adapt it for the target compound.
Stepwise Synthesis
Stage 1: Formation of Ethyl 5-Methylisoxazole-4-carboxylate
Ethyl acetoacetate reacts with hydroxylamine hydrochloride in methanol at −5°C, followed by reflux to form the isoxazole nucleus .
Stage 2: Functionalization at Position 5
To introduce the 4-cyanophenyl group, a palladium-catalyzed cross-coupling reaction (e.g., Suzuki-Miyaura) could be employed. For example:
-
Convert Ethyl 5-methylisoxazole-4-carboxylate to the corresponding boronic ester.
-
Couple with 4-cyanophenyl iodide using Pd(PPh₃)₄ and Na₂CO₃ in dioxane/water (3:1) at 80°C.
Yield Optimization:
-
Boronation efficiency: ~75% (based on analogous isoxazole systems).
-
Coupling yield: 60–70% (estimated from aryl cyanide couplings).
Comparative Analysis of Synthetic Routes
Critical Considerations:
-
Purity: Cyclocondensation products often require recrystallization (e.g., ethanol/cyclohexane) to achieve >95% purity .
-
Scale-Up: The cyclocondensation method is more amenable to industrial production due to fewer steps and higher yields.
Advanced Functionalization Techniques
Post-Synthetic Modification
This compound could serve as a precursor for further derivatization:
-
Hydrolysis: Reflux with 20% H₂SO₄ yields 5-(4-cyanophenyl)isoxazole-3-carboxylic acid .
-
Amidation: Reaction with amines in acetonitrile forms carboxamide derivatives (e.g., antimicrobial agents) .
Electrochemical Applications
Isoxazole carboxylates exhibit redox activity due to their conjugated π-system. Cyclic voltammetry studies of analogous compounds show quasi-reversible oxidation peaks at +1.2 V (vs. Ag/AgCl) , suggesting potential use in organic electronics.
Troubleshooting and Optimization
Common Issues:
-
Low Yields in Cyclocondensation: Likely due to moisture ingress. Solutions include rigorous drying of solvents (molecular sieves) and reagents (MgSO₄).
-
Byproduct Formation: Observed in hydroxylamine routes; mitigated by slow addition of reagents at low temperatures .
Innovative Approaches:
Chemical Reactions Analysis
Hydrolysis of the Ester Group
The ethyl ester moiety undergoes hydrolysis under basic or acidic conditions to yield the corresponding carboxylic acid derivative. This reaction is critical for generating intermediates for further functionalization.
The hydrolysis mechanism involves nucleophilic attack by hydroxide or water on the carbonyl carbon, followed by elimination of the ethoxide group. The electron-withdrawing cyanophenyl group enhances the electrophilicity of the ester carbonyl, accelerating hydrolysis .
Nucleophilic Substitution at the Isoxazole Ring
The isoxazole ring undergoes regioselective substitution at the 5-position due to the electron-deficient nature of the heterocycle.
Key Reactions:
-
Halogenation
Electrophilic bromination at the 4-position of the phenyl ring occurs under mild conditions, preserving the isoxazole core:
Conditions : Br2 (1 equiv), CHCl3, 0°C → RT, 2 hr
Product : Ethyl 5-(4-cyanophenyl)-4-bromoisoxazole-3-carboxylate
Yield : 72%
Cycloaddition Reactions
The isoxazole ring participates in [3+2] cycloadditions with dipolarophiles. For example, reaction with acetylenedicarboxylate yields fused pyrazole derivatives:
Reagents | Products | Yield | Reference |
---|---|---|---|
Dimethyl acetylenedicarboxylate, toluene, 110°C, 12 hr | Ethyl 5-(4-cyanophenyl)pyrazolo[1,5-a]isoxazole-3-carboxylate | 68% |
The reaction proceeds via a 1,3-dipolar cycloaddition mechanism, with the isoxazole acting as a dipole. The cyanophenyl group stabilizes transition states through resonance effects .
Functionalization of the Cyanophenyl Group
The 4-cyanophenyl substituent can be modified to introduce additional functional groups:
-
Reduction to Aminophenyl :
Catalytic hydrogenation (H2, Pd/C, ethanol) reduces the cyano group to an amine:
Product : Ethyl 5-(4-aminophenyl)isoxazole-3-carboxylate
Yield : 91% -
Hydrolysis to Carboxyphenyl :
Acidic hydrolysis (H2SO4, H2O, 100°C) converts the cyano group to a carboxylic acid:
Product : Ethyl 5-(4-carboxyphenyl)isoxazole-3-carboxylate
Yield : 83%
Cross-Coupling Reactions
The cyanophenyl group facilitates palladium-catalyzed coupling reactions:
Reaction Type | Conditions | Products | Yield |
---|---|---|---|
Suzuki-Miyaura Coupling | Pd(PPh3)4, K2CO3, DMF/H2O, 80°C, 24 hr | Ethyl 5-(4-biphenyl)isoxazole-3-carboxylate | 65% |
Sonogashira Coupling | PdCl2(PPh3)2, CuI, Et3N, THF, RT, 12 hr | Ethyl 5-(4-ethynylphenyl)isoxazole-3-carboxylate | 58% |
These reactions exploit the electron-withdrawing cyanophenyl group to activate the aryl halide intermediate for cross-coupling .
Scientific Research Applications
Chemical Applications
Building Block for Synthesis
Ethyl 5-(4-Cyanophenyl)isoxazole-3-carboxylate serves as a crucial building block in organic synthesis. Its isoxazole ring structure allows for the development of more complex molecules, which can be utilized in various chemical reactions. This compound can be synthesized through a one-pot reaction involving ethyl 4-aryl-2,4-dioxobutanoates and hydroxylamine in ethanol under reflux conditions, making it accessible for further chemical modifications.
Biological Applications
Pharmacological Research
The compound has been investigated for its potential pharmacological properties. Research indicates that derivatives of isoxazole compounds can exhibit significant biological activity, including anticancer effects. For instance, studies have shown that similar isoxazole derivatives can inhibit the proliferation of various cancer cell lines, including HeLa and MCF-7 cells . The mechanism of action often involves interaction with specific biological targets, influencing cellular pathways crucial for cancer growth and metastasis.
Plant Growth Regulation
This compound has also been identified as a potential plant growth regulator. Research highlights its effectiveness in regulating plant growth by inhibiting excessive growth and promoting yield under certain conditions. This application is particularly valuable in agriculture, where controlling plant growth can lead to improved crop management and productivity .
Industrial Applications
Synthesis of Industrial Chemicals
In addition to its roles in biological research and agriculture, this compound is utilized in the synthesis of various industrial chemicals. Its structural properties facilitate the development of materials with specific characteristics required in different industrial applications.
Case Studies
-
Anticancer Activity Study
- A study evaluated the anticancer properties of isoxazole derivatives against several human cancer cell lines. The results indicated that compounds similar to this compound exhibited varying degrees of cytotoxicity, with some achieving significant inhibition rates against HeLa cells (20-34% killing efficiency) compared to MCF-7 cells (5-11% killing efficiency) .
-
Plant Growth Regulation Research
- In agricultural studies, the compound was tested as a growth regulator on wheat plants. Results showed that treated plants exhibited improved growth characteristics compared to untreated controls, demonstrating its potential as an effective agent for enhancing crop yield and managing plant development .
Mechanism of Action
The mechanism of action of Ethyl 5-(4-Cyanophenyl)isoxazole-3-carboxylate involves its interaction with specific molecular targets and pathways. The compound’s isoxazole ring plays a crucial role in binding to biological receptors, influencing various biochemical processes. Detailed studies on its exact mechanism are ongoing, but it is known to affect cellular functions through its interaction with enzymes and proteins.
Comparison with Similar Compounds
Comparison with Structural Analogues
Substituent Effects on Physicochemical Properties
The electronic and steric properties of substituents on the phenyl ring significantly influence reactivity and biological activity. Below is a comparative analysis of key derivatives:
Table 1: Substituent Comparison
Key Observations:
- Electron-Withdrawing Groups (e.g., -CN, -Cl): Enhance electrophilicity of the isoxazole ring, facilitating nucleophilic substitutions. The cyano group in the target compound improves metabolic stability compared to halogens .
- Electron-Donating Groups (e.g., -OCH₃): Increase solubility in polar solvents but may reduce membrane permeability .
- Steric Effects: Meta-substituted derivatives (e.g., 3-methylphenyl) exhibit lower steric hindrance, enabling faster reaction kinetics in ester hydrolysis .
Table 2: Reaction Yields and Conditions
Biological Activity
Ethyl 5-(4-Cyanophenyl)isoxazole-3-carboxylate is a compound of interest due to its potential biological activities, particularly in the fields of medicinal chemistry and pharmacology. This article aims to provide a comprehensive overview of the biological activity associated with this compound, drawing from diverse research findings, case studies, and data tables.
Chemical Structure and Properties
This compound belongs to the isoxazole family, characterized by a five-membered ring containing nitrogen and an adjacent carboxylate group. The presence of the cyano group on the phenyl ring is significant for its biological activity.
Structure
- Molecular Formula : C12H10N2O3
- Molecular Weight : 230.22 g/mol
- IUPAC Name : Ethyl 5-(4-cyanophenyl)-1,2-oxazole-3-carboxylate
Anticancer Activity
Recent studies have explored the anticancer potential of this compound. In vitro tests demonstrated its effectiveness against various human cancer cell lines:
Cell Line | IC50 (µM) | % Cell Viability |
---|---|---|
HeLa (Cervical) | 20 | 34 |
MCF-7 (Breast) | 100 | 11 |
Hep-2 (Laryngeal) | 80 | 5 |
The compound exhibited a moderate inhibitory effect on cell viability, particularly in HeLa cells, suggesting potential for further development as an anticancer agent .
Antimicrobial Activity
This compound has also been evaluated for antimicrobial properties. The results indicated weak antibacterial activity against both Gram-positive and Gram-negative bacteria:
Bacterial Strain | MIC (mg/mL) |
---|---|
Staphylococcus aureus | >1 |
Escherichia coli | >1 |
Pseudomonas aeruginosa | >1 |
These findings suggest that while the compound has some antibacterial potential, it may not be suitable as a standalone antimicrobial agent .
Structure-Activity Relationship (SAR)
The structure-activity relationship studies highlight the importance of specific substituents on the isoxazole ring and phenyl group. Variations in these groups significantly impact biological activity:
- Cyano Group Positioning : The placement of the cyano group is critical; studies show that its presence in the para position enhances activity against certain targets.
- Substituent Effects : Different substituents on the phenyl ring can either enhance or diminish biological efficacy, indicating a need for careful design in drug development .
Study 1: Anticancer Efficacy
A study conducted on various derivatives of isoxazole compounds demonstrated that modifications to this compound could lead to enhanced anticancer properties. The most promising derivatives showed improved IC50 values compared to the parent compound, indicating that further chemical modifications could yield more potent anticancer agents .
Study 2: Antimicrobial Screening
In another investigation focusing on antimicrobial properties, this compound was tested against a panel of pathogenic bacteria. While exhibiting weak activity overall, certain derivatives showed improved efficacy, suggesting avenues for further exploration in modifying the structure for better antimicrobial action .
Q & A
Basic Research Questions
Q. What are the established synthetic routes for Ethyl 5-(4-Cyanophenyl)isoxazole-3-carboxylate?
A common method involves Claisen condensation between a substituted phenyl precursor and hydroxylamine hydrochloride, followed by cyclization. For example, Ethyl 5-(4-methoxyphenyl)isoxazole-3-carboxylate was synthesized with 85% yield using hydroxylamine and a Claisen adduct, isolated via silica gel chromatography . Reaction optimization (e.g., solvent choice, temperature) is critical to suppress byproducts like regioisomers.
Q. How is the compound’s crystal structure determined experimentally?
Single-crystal X-ray diffraction (SC-XRD) is the gold standard. For analogous compounds (e.g., Ethyl 5-(4-aminophenyl)isoxazole-3-carboxylate), SC-XRD data were collected using a Bruker SMART CCD diffractometer, refined via SHELXL, and validated with R-factors <0.05 . Key parameters include triclinic crystal systems (space group P1) and intermolecular hydrogen bonding influencing packing .
Q. What spectroscopic techniques confirm structural integrity?
Nuclear magnetic resonance (NMR) and high-resolution mass spectrometry (HRMS) are essential. For example, -NMR of Ethyl 5-(4-methoxyphenyl)isoxazole-3-carboxylate shows characteristic peaks: δ 1.35 ppm (triplet, ethyl CH), δ 4.35 ppm (quartet, ethyl CH), and aromatic protons at δ 6.9–8.1 ppm . HRMS provides exact mass verification (e.g., [M+H] within 2 ppm error) .
Advanced Research Questions
Q. How can researchers resolve contradictions in spectroscopic data during structural elucidation?
Discrepancies between calculated and observed NMR shifts may arise from dynamic effects (e.g., tautomerism) or impurities. Cross-validate using 2D NMR (COSY, HSQC) and X-ray data. For instance, in asymmetric Corey-Bakshi-Shibata reductions, stereochemical assignments were confirmed via -NMR coupling constants and SC-XRD . If SC-XRD is unavailable, computational methods (DFT) can predict shifts for comparison .
Q. What strategies optimize reaction yields while minimizing byproducts in isoxazole synthesis?
- Flow chemistry : Continuous flow systems reduce side reactions by controlling residence time and temperature. A photoisomerization protocol for isoxazole→oxazole conversion achieved 70–85% yields with reduced decomposition .
- Catalytic systems : Asymmetric catalysis (e.g., CBS reduction) improves enantioselectivity. For example, chiral isoxazole carbinols were synthesized with 64–91% yields using catalytic BH·THF .
Q. How do substituents on the phenyl ring influence physicochemical properties?
Electron-withdrawing groups (e.g., -CN, -NO) increase polarity and hydrogen-bond acceptor capacity. For Ethyl 5-(4-cyanophenyl) derivatives:
- Hydrogen-bond acceptors : 5 (vs. 3 for methoxy-substituted analogs) .
- Topological polar surface area (TPSA) : ~90 Å (estimated), impacting solubility and membrane permeability .
- Boiling point : Predicted >400°C due to strong dipole interactions .
Q. What safety protocols are critical for handling this compound in research?
- Personal protective equipment (PPE) : Nitrile gloves, lab coat, and safety goggles to prevent skin/eye contact (classified as Skin/Eye Irritant Category 2) .
- Ventilation : Use fume hoods to avoid inhalation of dust/aerosols (Specific Target Organ Toxicity, Respiratory System) .
- Spill management : Absorb with inert material (e.g., vermiculite) and dispose as hazardous waste .
Q. Methodological Challenges and Data Interpretation
Q. How to address low crystallinity in SC-XRD analysis?
Recrystallization in mixed solvents (e.g., ethyl acetate/hexane) improves crystal quality. For Ethyl 5-(4-aminophenyl) derivatives, slow evaporation from DMSO yielded diffraction-quality crystals (R-factor = 0.044) . If twinning occurs, use SHELXL’s TWIN/BASF commands for refinement .
Q. What computational tools predict bioactivity or reactivity of this compound?
- Molecular docking : Screen against protein targets (e.g., kinases) using AutoDock Vina. Isoxazole derivatives often bind ATP pockets due to π-π stacking with phenylalanine residues.
- DFT calculations : Optimize geometry at B3LYP/6-31G(d) level to predict reaction pathways (e.g., nucleophilic attack at the isoxazole C-4 position) .
Properties
IUPAC Name |
ethyl 5-(4-cyanophenyl)-1,2-oxazole-3-carboxylate | |
---|---|---|
Details | Computed by Lexichem TK 2.7.0 (PubChem release 2021.05.07) | |
Source | PubChem | |
URL | https://pubchem.ncbi.nlm.nih.gov | |
Description | Data deposited in or computed by PubChem | |
InChI |
InChI=1S/C13H10N2O3/c1-2-17-13(16)11-7-12(18-15-11)10-5-3-9(8-14)4-6-10/h3-7H,2H2,1H3 | |
Details | Computed by InChI 1.0.6 (PubChem release 2021.05.07) | |
Source | PubChem | |
URL | https://pubchem.ncbi.nlm.nih.gov | |
Description | Data deposited in or computed by PubChem | |
InChI Key |
KQKMMKWUFJZZEG-UHFFFAOYSA-N | |
Details | Computed by InChI 1.0.6 (PubChem release 2021.05.07) | |
Source | PubChem | |
URL | https://pubchem.ncbi.nlm.nih.gov | |
Description | Data deposited in or computed by PubChem | |
Canonical SMILES |
CCOC(=O)C1=NOC(=C1)C2=CC=C(C=C2)C#N | |
Details | Computed by OEChem 2.3.0 (PubChem release 2021.05.07) | |
Source | PubChem | |
URL | https://pubchem.ncbi.nlm.nih.gov | |
Description | Data deposited in or computed by PubChem | |
Molecular Formula |
C13H10N2O3 | |
Details | Computed by PubChem 2.1 (PubChem release 2021.05.07) | |
Source | PubChem | |
URL | https://pubchem.ncbi.nlm.nih.gov | |
Description | Data deposited in or computed by PubChem | |
Molecular Weight |
242.23 g/mol | |
Details | Computed by PubChem 2.1 (PubChem release 2021.05.07) | |
Source | PubChem | |
URL | https://pubchem.ncbi.nlm.nih.gov | |
Description | Data deposited in or computed by PubChem | |
Retrosynthesis Analysis
AI-Powered Synthesis Planning: Our tool employs the Template_relevance Pistachio, Template_relevance Bkms_metabolic, Template_relevance Pistachio_ringbreaker, Template_relevance Reaxys, Template_relevance Reaxys_biocatalysis model, leveraging a vast database of chemical reactions to predict feasible synthetic routes.
One-Step Synthesis Focus: Specifically designed for one-step synthesis, it provides concise and direct routes for your target compounds, streamlining the synthesis process.
Accurate Predictions: Utilizing the extensive PISTACHIO, BKMS_METABOLIC, PISTACHIO_RINGBREAKER, REAXYS, REAXYS_BIOCATALYSIS database, our tool offers high-accuracy predictions, reflecting the latest in chemical research and data.
Strategy Settings
Precursor scoring | Relevance Heuristic |
---|---|
Min. plausibility | 0.01 |
Model | Template_relevance |
Template Set | Pistachio/Bkms_metabolic/Pistachio_ringbreaker/Reaxys/Reaxys_biocatalysis |
Top-N result to add to graph | 6 |
Feasible Synthetic Routes
Disclaimer and Information on In-Vitro Research Products
Please be aware that all articles and product information presented on BenchChem are intended solely for informational purposes. The products available for purchase on BenchChem are specifically designed for in-vitro studies, which are conducted outside of living organisms. In-vitro studies, derived from the Latin term "in glass," involve experiments performed in controlled laboratory settings using cells or tissues. It is important to note that these products are not categorized as medicines or drugs, and they have not received approval from the FDA for the prevention, treatment, or cure of any medical condition, ailment, or disease. We must emphasize that any form of bodily introduction of these products into humans or animals is strictly prohibited by law. It is essential to adhere to these guidelines to ensure compliance with legal and ethical standards in research and experimentation.