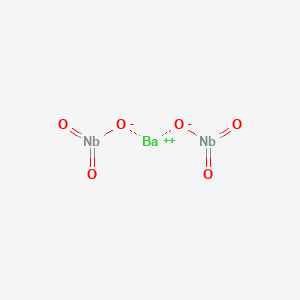
Barium(2+);oxido(dioxo)niobium
- Click on QUICK INQUIRY to receive a quote from our team of experts.
- With the quality product at a COMPETITIVE price, you can focus more on your research.
Overview
Description
Barium(2+);oxido(dioxo)niobium, systematically named barium niobate, is an inorganic compound with the molecular formula BaNb₂O₆ (CAS: 12009-14-2) . Its structure consists of barium cations (Ba²⁺) and niobate anions (NbO₃⁻), arranged in a perovskite-related lattice. The InChI descriptor for this compound is InChI=1/Ba.2Nb.6O/q+2;;;;;;;2*-1/rBa.2NbO3/c;2*2-1(3)4/q+2;2*-1, reflecting its coordination environment . Barium niobate is utilized in electronic ceramics, catalysts, and dielectric materials due to its high thermal stability and tunable electrical properties .
Preparation Methods
Synthetic Routes and Reaction Conditions
The synthesis of barium niobium oxide can be achieved through various methods, including solid-state reactions, sol-gel processes, and hydrothermal synthesis. One common method involves the solid-state reaction of barium carbonate (BaCO₃) and niobium pentoxide (Nb₂O₅) at high temperatures. The reaction typically occurs at temperatures ranging from 900°C to 1200°C in an oxygen-rich environment to ensure complete oxidation of niobium.
Another method involves the sol-gel process, where barium and niobium precursors are dissolved in a solvent to form a homogeneous solution. This solution undergoes gelation, followed by drying and calcination at high temperatures to form the desired oxide compound. The sol-gel method allows for better control over the particle size and morphology of the final product.
Industrial Production Methods
In industrial settings, the production of barium niobium oxide often involves large-scale solid-state reactions. The raw materials, such as barium carbonate and niobium pentoxide, are mixed in stoichiometric ratios and subjected to high-temperature calcination in rotary kilns or furnaces. The process parameters, including temperature, pressure, and reaction time, are carefully controlled to ensure the formation of a pure and homogeneous product.
Chemical Reactions Analysis
Types of Reactions
Barium niobium oxide undergoes various chemical reactions, including oxidation, reduction, and substitution reactions. These reactions are influenced by the oxidation state of niobium and the presence of reactive oxygen species.
Common Reagents and Conditions
Oxidation Reactions: Barium niobium oxide can undergo oxidation reactions in the presence of strong oxidizing agents, such as hydrogen peroxide (H₂O₂) or potassium permanganate (KMnO₄). These reactions typically occur under acidic or basic conditions and result in the formation of higher oxidation state niobium compounds.
Reduction Reactions: Reduction of barium niobium oxide can be achieved using reducing agents like hydrogen gas (H₂) or sodium borohydride (NaBH₄). These reactions are often carried out at elevated temperatures and result in the formation of lower oxidation state niobium compounds.
Substitution Reactions: Substitution reactions involve the replacement of oxygen atoms in the compound with other anions, such as fluorine or chlorine. These reactions can be carried out using halogenating agents like hydrofluoric acid (HF) or chlorine gas (Cl₂).
Major Products Formed
The major products formed from these reactions depend on the specific reagents and conditions used. For example, oxidation reactions may yield niobium pentoxide (Nb₂O₅), while reduction reactions may produce niobium dioxide (NbO₂) or niobium monoxide (NbO). Substitution reactions can result in the formation of niobium halides, such as niobium pentafluoride (NbF₅) or niobium pentachloride (NbCl₅).
Scientific Research Applications
Barium niobium oxide has a wide range of scientific research applications due to its unique properties. Some of the key applications include:
Mechanism of Action
The mechanism of action of barium niobium oxide in various applications is primarily attributed to its unique electronic structure and surface properties. In energy storage applications, the compound’s ability to undergo reversible redox reactions allows for efficient charge and discharge cycles. The presence of niobium in a high oxidation state facilitates the transfer of electrons and ions, enhancing the overall performance of the material.
In catalysis, the active sites on the surface of barium niobium oxide promote the adsorption and activation of reactant molecules, leading to increased reaction rates. The compound’s high surface area and porosity also contribute to its catalytic efficiency.
Comparison with Similar Compounds
Potassium Niobate (KNbO₃)
Potassium niobate (KNbO₃) shares a perovskite structure (ABO₃) with barium niobate but differs in cation composition. Key comparisons include:
- Crystal Structure : KNbO₃ adopts a classic perovskite lattice, whereas BaNb₂O₆ exhibits a layered or distorted perovskite structure due to the higher Nb:Ba ratio .
- Applications: KNbO₃ is prominent in nonlinear optics and piezoelectric devices, whereas BaNb₂O₆ is favored in dielectric resonators and microwave ceramics .
- Electrical Properties : KNbO₃ displays ferroelectricity below its Curie temperature (~435°C), while BaNb₂O₆ shows paraelectric behavior with a high dielectric constant (>100) at room temperature .
Table 1: Structural and Functional Comparison of Niobates
Compound | Formula | Structure | Key Applications | Notable Properties |
---|---|---|---|---|
Barium niobate | BaNb₂O₆ | Layered perovskite | Dielectric ceramics | High dielectric constant (>100) |
Potassium niobate | KNbO₃ | Cubic perovskite | Piezoelectric sensors | Ferroelectric, nonlinear optical |
Barium Titanate (BaTiO₃) and Niobium-Doped Variants
Barium titanate (BaTiO₃) is a well-studied perovskite oxide with distinct differences from BaNb₂O₆:
- Crystal Phase : BaTiO₃ transitions between tetragonal (ferroelectric) and cubic (paraelectric) phases, whereas BaNb₂O₆ maintains a stable paraelectric phase .
- PTCR Effect: Niobium-doped BaTiO₃ (e.g., BaTiO₃:Nb) exhibits a pronounced positive temperature coefficient of resistivity (PTCR), making it ideal for thermistors. In contrast, BaNb₂O₆ lacks this property but offers superior dielectric loss characteristics .
- Synthesis : Both compounds are synthesized via solid-state reactions, but Nb doping in BaTiO₃ enhances grain boundary barrier layers, critical for PTCR behavior .
Table 2: Electrical Behavior of Barium-Based Perovskites
Compound | PTCR Effect | Dielectric Constant (1 kHz) | Primary Use |
---|---|---|---|
BaTiO₃ (undoped) | No | ~1,200 | Capacitors |
BaTiO₃:Nb | Yes | ~5,000 | Thermistors, sensors |
BaNb₂O₆ | No | ~100 | Microwave ceramics |
Other Niobates and Oxides
- Strontium Niobate (SrNb₂O₆) : Shares a similar layered structure with BaNb₂O₆ but shows higher ionic conductivity, suited for solid oxide fuel cells .
- Cadmium Oxalate (CdC₂O₄) : While structurally distinct (oxalate ligand vs. niobate), CdC₂O₄ highlights the diversity in metal-oxygen coordination chemistry, though it lacks electronic applications .
Q & A
Basic Research Questions
Q. What are the optimal synthesis conditions for barium niobium oxide (BaNb₂O₆) to achieve phase purity?
- Methodological Answer : Synthesis typically involves solid-state calcination of stoichiometric mixtures of BaCO₃ and Nb₂O₅ at 1100–1300°C under controlled oxygen flow. Phase purity is confirmed via X-ray diffraction (XRD), where lattice parameters (e.g., hexagonal structure with space group P6₃/mmc) should align with literature values. Oxygen partial pressure must be optimized to prevent oxygen deficiency, as Nb⁵⁺ stability depends on oxidizing conditions .
Q. Which analytical techniques are critical for characterizing the structural and electronic properties of barium niobium oxide?
- Methodological Answer :
- XRD : Determines crystallinity and phase composition.
- XPS : Surface-sensitive analysis to confirm Nb⁵⁺ oxidation state and Ba²⁺ coordination. Note that XPS has limited bulk penetration (~10–20 atomic layers) and should be complemented with bulk techniques .
- TEM/EDS : Maps elemental distribution (e.g., verifying uniform Nb doping in bulk particles) .
Advanced Research Questions
Q. How does niobium doping influence cation mixing (Li⁺/Ni²⁺) in layered oxide cathodes, and why do conflicting reports exist in the literature?
- Methodological Answer : Nb⁵⁺ doping in transition metal (TM) sites reduces Li⁺/Ni²⁺ mixing by creating lithium vacancies, as charge balance requires compensating for Nb⁵⁺ substitution. Contradictions arise from differing synthesis conditions (e.g., calcination time/temperature) and characterization methods. For example, XPS may suggest reduced Ni²⁺ content, while bulk-sensitive neutron diffraction reveals higher Ni²⁺ retention .
Q. How can researchers resolve discrepancies in reported Ni²⁺/Ni³⁺ ratios in Nb-doped cathodes?
- Methodological Answer : Combine surface (XPS) and bulk (Mössbauer spectroscopy, neutron diffraction) techniques. For instance, EDS mapping of cross-sectioned particles confirms uniform Nb distribution, addressing surface-bulk disparities. Statistical analysis of defect concentrations (e.g., box-and-whisker plots) can quantify variability across studies .
Q. What is the role of oxygen stoichiometry in stabilizing Nb⁵⁺ in barium niobium oxide, and how does it impact electrochemical performance?
- Methodological Answer : Oxygen vacancies destabilize Nb⁵⁺, leading to partial reduction to Nb⁴⁺. Controlled oxygen flow during synthesis maintains the Nb₂O₅ ratio (1:2.5 metal-to-oxygen), critical for doping efficiency. Incomplete oxidation during calcination may leave residual Ni²⁺, affecting charge capacity in cathodes .
Q. What advanced computational methods validate the electronic structure of barium niobium oxide?
- Methodological Answer : Density functional theory (DFT) with generalized gradient approximation (GGA) predicts lattice constants and bandgap variations. For example, GGA corrects LSD underestimation of lattice parameters in oxides and confirms Nb–O bond strength, aligning with experimental XPS data .
Q. Data Contradiction Analysis
Q. Why do some studies report improved cycle retention in Nb-doped cathodes while others observe capacity fading?
- Methodological Answer : Discrepancies stem from doping concentration thresholds. At ≤1 wt% Nb, cycle retention improves due to suppressed phase transitions. Above 3 wt%, excess Nb forms insulating Nb₂O₅ phases, increasing impedance. Electrochemical impedance spectroscopy (EIS) and TEM of cycled electrodes differentiate bulk vs. surface degradation .
Q. Methodological Tables
Properties
IUPAC Name |
barium(2+);oxido(dioxo)niobium |
Source
|
---|---|---|
Details | Computed by LexiChem 2.6.6 (PubChem release 2019.06.18) | |
Source | PubChem | |
URL | https://pubchem.ncbi.nlm.nih.gov | |
Description | Data deposited in or computed by PubChem | |
InChI |
InChI=1S/Ba.2Nb.6O/q+2;;;;;;;2*-1 |
Source
|
Details | Computed by InChI 1.0.5 (PubChem release 2019.06.18) | |
Source | PubChem | |
URL | https://pubchem.ncbi.nlm.nih.gov | |
Description | Data deposited in or computed by PubChem | |
InChI Key |
FKKSQMDUUDBSGH-UHFFFAOYSA-N |
Source
|
Details | Computed by InChI 1.0.5 (PubChem release 2019.06.18) | |
Source | PubChem | |
URL | https://pubchem.ncbi.nlm.nih.gov | |
Description | Data deposited in or computed by PubChem | |
Canonical SMILES |
[O-][Nb](=O)=O.[O-][Nb](=O)=O.[Ba+2] |
Source
|
Details | Computed by OEChem 2.1.5 (PubChem release 2019.06.18) | |
Source | PubChem | |
URL | https://pubchem.ncbi.nlm.nih.gov | |
Description | Data deposited in or computed by PubChem | |
Molecular Formula |
BaNb2O6 |
Source
|
Details | Computed by PubChem 2.1 (PubChem release 2019.06.18) | |
Source | PubChem | |
URL | https://pubchem.ncbi.nlm.nih.gov | |
Description | Data deposited in or computed by PubChem | |
Molecular Weight |
419.14 g/mol |
Source
|
Details | Computed by PubChem 2.1 (PubChem release 2021.05.07) | |
Source | PubChem | |
URL | https://pubchem.ncbi.nlm.nih.gov | |
Description | Data deposited in or computed by PubChem | |
Disclaimer and Information on In-Vitro Research Products
Please be aware that all articles and product information presented on BenchChem are intended solely for informational purposes. The products available for purchase on BenchChem are specifically designed for in-vitro studies, which are conducted outside of living organisms. In-vitro studies, derived from the Latin term "in glass," involve experiments performed in controlled laboratory settings using cells or tissues. It is important to note that these products are not categorized as medicines or drugs, and they have not received approval from the FDA for the prevention, treatment, or cure of any medical condition, ailment, or disease. We must emphasize that any form of bodily introduction of these products into humans or animals is strictly prohibited by law. It is essential to adhere to these guidelines to ensure compliance with legal and ethical standards in research and experimentation.