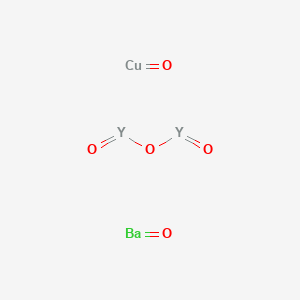
Yttrium barium copper oxide
Overview
Description
Yttrium barium copper oxide (YBCO) is a high-temperature superconducting (HTS) material discovered in 1987, with a critical temperature (Tc) of ~90 K, surpassing the boiling point of liquid nitrogen (77 K). This breakthrough enabled practical applications due to cheaper cooling requirements compared to liquid helium-dependent superconductors .
Preparation Methods
Synthetic Routes and Reaction Conditions: Yttrium barium copper oxide can be synthesized by heating a mixture of metal carbonates at temperatures between 1000 to 1300 K. The reaction involves yttrium carbonate, barium carbonate, and copper carbonate, which react to form this compound and carbon dioxide . Modern synthesis methods use the corresponding oxides and nitrates instead of carbonates .
Industrial Production Methods: Industrial production of this compound often involves the trifluoroacetic acid-metal-organic deposition (TFA-MOD) method. This method includes preparing a precursor solution by mixing yttrium acetate, barium acetate, and copper acetate in trifluoroacetic acid, followed by thermal treatment to form the superconducting thin film .
Chemical Reactions Analysis
3.1. Phase Formation
The formation of different phases during synthesis significantly impacts the superconducting properties of YBCO. The primary phases include:
-
Orthorhombic Phase : This phase is essential for superconductivity and is stabilized by proper oxygen content.
-
Monoclinic Phase : Often formed as a by-product during synthesis, this phase does not exhibit superconductivity and can degrade performance.
3.2. Doping Effects
Doping with elements such as aluminum or iron can enhance the superconducting properties of YBCO by modifying its microstructure and thermal stability:
-
Aluminum doping has been shown to lower the activation energy required for phase transitions during heating, leading to improved synthesis efficiency.
-
Iron microparticles can promote the formation of the desired superconducting phase while preventing the formation of undesirable phases like barium carbonate .
Characterization Techniques
Characterization of YBCO involves various analytical techniques to determine phase purity, microstructure, and superconducting properties:
-
X-ray Diffraction (XRD) : Used to identify crystalline phases and assess structural integrity.
-
Thermogravimetric Analysis (TGA) : Measures mass changes as a function of temperature to study thermal stability and decomposition processes.
-
Differential Thermal Analysis (DTA) : Provides insights into phase transitions during heating.
Scientific Research Applications
Electronics
YBCO's low surface resistance and high critical current density make it an excellent candidate for various electronic applications:
- Microwave Devices : YBCO is utilized in microwave technology due to its ability to maintain low losses at high frequencies. This application is particularly relevant in telecommunications and radar systems where signal integrity is crucial .
- Superconducting Quantum Interference Devices (SQUIDs) : These devices leverage the superconducting properties of YBCO for sensitive magnetic field measurements, making them invaluable in research and medical imaging technologies .
Energy Storage
YBCO plays a pivotal role in the development of superconducting magnetic energy storage systems (SMES):
- SMES Systems : These systems utilize YBCO coils to store energy in the magnetic field created by the flow of direct current. The efficiency of energy storage and rapid discharge capabilities make SMES suitable for stabilizing power grids and providing backup power during outages .
- Wind and Solar Energy Integration : As renewable energy sources become more prevalent, YBCO-based systems can help manage fluctuations in energy supply by storing excess energy generated during peak production times .
Medical Applications
In the medical field, YBCO's properties are harnessed for advanced imaging technologies:
- Magnetic Resonance Imaging (MRI) : Superconducting magnets made from YBCO are used to enhance MRI machines' performance, improving image resolution and reducing scan times .
- Magnetoencephalography (MEG) : This technique measures magnetic fields produced by neural activity. YBCO sensors provide higher sensitivity and accuracy compared to traditional sensors, facilitating better diagnosis of neurological conditions .
Advanced Materials
YBCO's unique characteristics enable its use in developing new materials with enhanced properties:
- Coatings for Aerospace : The lightweight and strong nature of YBCO makes it suitable for protective coatings in aerospace applications, enhancing durability while reducing weight .
- Composite Materials : Incorporating YBCO into composite materials can improve their electrical conductivity and thermal stability, making them suitable for various engineering applications .
Case Study 1: Microwave Applications
A study on the use of YBCO in microwave devices demonstrated that incorporating this superconductor significantly reduced signal loss compared to conventional materials. The research indicated that devices utilizing YBCO could operate effectively at frequencies above 100 GHz, showcasing its potential in next-generation communication systems .
Case Study 2: Energy Storage Systems
In a practical implementation of SMES using YBCO, researchers developed a prototype system that successfully stabilized a local power grid during peak demand periods. The system was able to discharge stored energy within milliseconds, proving effective in maintaining grid stability during fluctuations caused by renewable energy sources .
Mechanism of Action
Yttrium barium copper oxide is part of a broader group of rare-earth barium copper oxides, which include compounds with other rare earth elements such as lanthanum, samarium, neodymium, gadolinium, and europium . These compounds share similar high-temperature superconducting properties but differ in their critical temperatures and structural details. For example, lanthanum barium copper oxide becomes superconducting at 35 K, while this compound has a higher critical temperature of 93 K .
Comparison with Similar Compounds
Crystal Structure and Composition
YBCO adopts a layered perovskite-derived structure (Figure 3c in ), where yttrium ions occupy the A-site, barium and copper ions form the B-site, and oxygen vacancies (δ ≈ 0.3–0.5) are critical for superconductivity . The structure comprises two conductive CuO₂ planes separated by yttrium atoms (3.2 Å spacing), facilitating anisotropic current flow . Oxygen deficiency in the Cu-O chains stabilizes the material’s superconducting phase .
Lanthanum Barium Copper Oxide (La-Ba-Cu-O)
Discovery: The La-Ba-Cu-O system (Tc ≈ 35 K) was the first copper-oxide HTS discovered in 1986, preceding YBCO . Structure: Shares a perovskite framework but lacks the layered CuO₂ planes of YBCO, resulting in lower Tc .
Bismuth Strontium Calcium Copper Oxide (Bi₂Sr₂Ca₂Cu₃O₁₀₊δ, BSCCO)
Critical Temperature : BSCCO exhibits higher Tc (~110 K) but requires complex synthesis due to its multi-layered structure with alternating Bi-O and CuO₂ planes .
Structure : A "2212" or "2223" phase structure with variable oxygen content, enabling flexibility in doping but complicating phase purity .
Applications : Used in high-field magnets and superconducting tapes, though YBCO dominates commercial markets due to better mechanical stability and current-carrying capacity .
Thallium-Based Superconductors (Tl-Ba-Ca-Cu-O)
Critical Temperature : Tl₂Ba₂Ca₂Cu₃O₁₀ (Tc ~125 K) holds the record among cuprates but is rarely used due to thallium’s toxicity and synthesis challenges .
Research Findings and Performance Metrics
Defect and Irradiation Tolerance
YBCO’s defect dynamics under heavy-ion irradiation are well-studied, with defects reducing Tc but enabling flux-pinning enhancements in thin films . BSCCO and La-Ba-Cu-O show less resilience to radiation-induced degradation .
Market and Industrial Relevance
YBCO holds ~40% of the superconducting materials market, driven by MRI and energy applications, while BSCCO caters to niche high-field uses . Additive manufacturing advancements are expanding YBCO’s utility in complex geometries .
Biological Activity
Yttrium barium copper oxide (YBCO), specifically YBaCuO, is a high-temperature superconductor (HTS) notable for its significant applications in various fields, including electronics, energy generation, and biomedical applications. Beyond its superconducting properties, recent studies have highlighted its biological activity, particularly its antibacterial properties and potential applications in nanomedicine.
Antibacterial Properties
Recent research has focused on the synthesis and evaluation of YBCO nanoparticles (NPs) for their antibacterial effectiveness. The sol-gel method has been employed to produce these nanoparticles, which were then tested against various bacterial strains.
Case Study: Antibacterial Efficacy
A study published in The Journal of Engineering and Exact Sciences investigated the antibacterial activity of YBCO NPs against four bacterial strains: Staphylococcus epidermidis, Methicillin-resistant Staphylococcus aureus (MRSA), Escherichia coli, and Pseudomonas aeruginosa. The results indicated that:
- Gram-positive bacteria (e.g., Staphylococcus epidermidis and MRSA) showed significant susceptibility to YBCO NPs at concentrations of 0.01 mg/ml to 0.1 mg/ml.
- Gram-negative bacteria exhibited minimal response to the same concentrations.
The study concluded that YBCO NPs could serve as a potential antibacterial agent, particularly against certain Gram-positive pathogens .
Cytotoxicity Studies
In addition to antibacterial activity, the cytotoxic effects of yttrium oxide nanoparticles (related to YBCO) have been investigated. A study assessed the size-dependent cytotoxicity of these nanoparticles on primary osteoblasts in vitro:
- Smaller yttrium oxide particles exhibited higher toxicity levels compared to larger particles.
- Mechanisms of cytotoxicity were linked to increased reactive oxygen species (ROS) generation, leading to cell damage .
Synthesis Methods
The synthesis of YBCO NPs typically involves:
- Sol-Gel Method : Utilizes precursors such as Yttrium Oxide (YO), Barium Carbonate (BaCO), and Copper Oxide (CuO). The process includes complexing agents like citric acid and polymerization agents like ethylene glycol .
Table 1: Synthesis Parameters for YBCO NPs
Component | Chemical Formula | Purity (%) |
---|---|---|
Yttrium Oxide | YO | 90 |
Barium Carbonate | BaCO | 99.99 |
Copper Oxide | CuO | 78-79 |
Citric Acid | CHO | - |
Ethylene Glycol | CHO | - |
Superconducting Properties and Biological Applications
YBCO's superconducting properties are influenced by its crystal structure and phase composition, which can be affected by factors such as oxygen content. Controlled synthesis methods allow for the production of structurally stable forms that retain both superconducting and biological functionalities.
Applications in Nanomedicine
YBCO's unique properties make it a candidate for various biomedical applications, including:
Q & A
Basic Research Questions
Q. What experimental methodologies are recommended for synthesizing high-purity YBCO samples?
- YBCO synthesis typically involves solid-state reactions using stoichiometric ratios of yttrium oxide (Y₂O₃), barium carbonate (BaCO₃), and copper oxide (CuO). Precursors are mixed, calcined at 900–950°C in oxygen-rich environments, and sintered to form the orthorhombic perovskite structure . Purity (>99.9%) is critical; contaminants like carbon residues degrade superconducting properties. Post-synthesis characterization via X-ray diffraction (XRD) and energy-dispersive X-ray spectroscopy (EDX) ensures phase purity and stoichiometric accuracy .
Q. How is the critical temperature (Tₐ) of YBCO measured reproducibly?
- Tₐ determination requires a cryogenic setup with four-probe resistivity measurements or magnetic susceptibility (e.g., SQUID magnetometry). A standardized protocol involves cooling YBCO in zero-field conditions, monitoring resistivity drops, and identifying the midpoint of the transition curve. Reproducibility hinges on sample homogeneity and oxygen stoichiometry control (e.g., annealing at 400–500°C in O₂ to optimize oxygen vacancies) .
Q. What structural features of YBCO underpin its superconducting properties?
- YBCO’s orthorhombic crystal structure (space group Pmmm) contains CuO₂ planes responsible for Cooper pair formation. Oxygen vacancies in the CuO chains modulate carrier density, directly affecting Tₐ. Synchrotron X-ray absorption spectroscopy (XAS) and neutron diffraction are used to map oxygen ordering and lattice distortions .
Advanced Research Questions
Q. How can researchers resolve contradictions in reported Tₐ values for YBCO thin films?
- Discrepancies arise from variations in thin-film fabrication (e.g., pulsed laser deposition vs. sputtering) and substrate-induced strain. To mitigate this, standardize growth parameters (e.g., substrate temperature: 700–800°C, oxygen partial pressure: 200–300 mTorr) and characterize strain effects via Raman spectroscopy or high-resolution XRD . Cross-referencing with transport measurements (e.g., radio-frequency impedance) validates intrinsic Tₐ .
Q. What advanced techniques optimize YBCO’s current-carrying capacity in applied magnetic fields?
- Flux pinning enhancement via nanoscale defect engineering (e.g., BaZrO₃ nanoparticle inclusion) improves critical current density (J꜀). Transmission electron microscopy (TEM) and magneto-optical imaging quantify defect density and flux dynamics . For high-field applications, measure J꜀ using Bean’s critical state model in varying magnetic field orientations .
Q. How do doping strategies (e.g., Ca²⁺ substitution) alter YBCO’s electronic structure?
- Doping at Y³⁺ or Ba²⁺ sites modifies hole concentration in CuO₂ planes. For example, Ca²⁺ substitution at Y³⁺ sites increases hole density, raising Tₐ but reducing structural stability. Use angle-resolved photoemission spectroscopy (ARPES) to track Fermi surface changes and muon spin rotation (μSR) to probe magnetic inhomogeneity .
Q. What methodologies address oxygen stoichiometry challenges in YBCO single crystals?
- Oxygen content (7−x in YBa₂Cu₃O₇₋ₓ) is controlled via post-annealing in O₂/N₂ atmospheres. Thermogravimetric analysis (TGA) monitors oxygen uptake/release, while extended X-ray fine structure (EXAFS) quantifies local oxygen coordination. Note that oxygen mobility decreases below 300°C, requiring slow cooling rates (~1°C/min) to avoid phase segregation .
Q. Methodological Best Practices
- Data Validation : Cross-validate electrical and magnetic measurements to distinguish intrinsic superconductivity from artifacts (e.g., filamentary pathways) .
- Sample Reproducibility : Document oxygen annealing history and sintering conditions to ensure batch consistency .
- Advanced Characterization : Pair macroscopic measurements (e.g., resistivity) with microscopic probes (e.g., scanning tunneling microscopy) to correlate structure-property relationships .
Properties
IUPAC Name |
oxobarium;oxocopper;oxo(oxoyttriooxy)yttrium | |
---|---|---|
Details | Computed by LexiChem 2.6.6 (PubChem release 2019.06.18) | |
Source | PubChem | |
URL | https://pubchem.ncbi.nlm.nih.gov | |
Description | Data deposited in or computed by PubChem | |
InChI |
InChI=1S/Ba.Cu.5O.2Y | |
Details | Computed by InChI 1.0.5 (PubChem release 2019.06.18) | |
Source | PubChem | |
URL | https://pubchem.ncbi.nlm.nih.gov | |
Description | Data deposited in or computed by PubChem | |
InChI Key |
OHBOMLDLKVPQKS-UHFFFAOYSA-N | |
Details | Computed by InChI 1.0.5 (PubChem release 2019.06.18) | |
Source | PubChem | |
URL | https://pubchem.ncbi.nlm.nih.gov | |
Description | Data deposited in or computed by PubChem | |
Canonical SMILES |
O=[Cu].O=[Y]O[Y]=O.O=[Ba] | |
Details | Computed by OEChem 2.1.5 (PubChem release 2019.06.18) | |
Source | PubChem | |
URL | https://pubchem.ncbi.nlm.nih.gov | |
Description | Data deposited in or computed by PubChem | |
Molecular Formula |
BaCuO5Y2 | |
Details | Computed by PubChem 2.1 (PubChem release 2019.06.18) | |
Source | PubChem | |
URL | https://pubchem.ncbi.nlm.nih.gov | |
Description | Data deposited in or computed by PubChem | |
Molecular Weight |
458.68 g/mol | |
Details | Computed by PubChem 2.1 (PubChem release 2021.05.07) | |
Source | PubChem | |
URL | https://pubchem.ncbi.nlm.nih.gov | |
Description | Data deposited in or computed by PubChem | |
Physical Description |
Black odorless powder; [MSDSonline] | |
Record name | Yttrium barium copper oxide | |
Source | Haz-Map, Information on Hazardous Chemicals and Occupational Diseases | |
URL | https://haz-map.com/Agents/8723 | |
Description | Haz-Map® is an occupational health database designed for health and safety professionals and for consumers seeking information about the adverse effects of workplace exposures to chemical and biological agents. | |
Explanation | Copyright (c) 2022 Haz-Map(R). All rights reserved. Unless otherwise indicated, all materials from Haz-Map are copyrighted by Haz-Map(R). No part of these materials, either text or image may be used for any purpose other than for personal use. Therefore, reproduction, modification, storage in a retrieval system or retransmission, in any form or by any means, electronic, mechanical or otherwise, for reasons other than personal use, is strictly prohibited without prior written permission. | |
CAS No. |
107539-20-8 | |
Record name | Yttrium barium copper oxide | |
Source | European Chemicals Agency (ECHA) | |
URL | https://echa.europa.eu/information-on-chemicals | |
Description | The European Chemicals Agency (ECHA) is an agency of the European Union which is the driving force among regulatory authorities in implementing the EU's groundbreaking chemicals legislation for the benefit of human health and the environment as well as for innovation and competitiveness. | |
Explanation | Use of the information, documents and data from the ECHA website is subject to the terms and conditions of this Legal Notice, and subject to other binding limitations provided for under applicable law, the information, documents and data made available on the ECHA website may be reproduced, distributed and/or used, totally or in part, for non-commercial purposes provided that ECHA is acknowledged as the source: "Source: European Chemicals Agency, http://echa.europa.eu/". Such acknowledgement must be included in each copy of the material. ECHA permits and encourages organisations and individuals to create links to the ECHA website under the following cumulative conditions: Links can only be made to webpages that provide a link to the Legal Notice page. | |
Disclaimer and Information on In-Vitro Research Products
Please be aware that all articles and product information presented on BenchChem are intended solely for informational purposes. The products available for purchase on BenchChem are specifically designed for in-vitro studies, which are conducted outside of living organisms. In-vitro studies, derived from the Latin term "in glass," involve experiments performed in controlled laboratory settings using cells or tissues. It is important to note that these products are not categorized as medicines or drugs, and they have not received approval from the FDA for the prevention, treatment, or cure of any medical condition, ailment, or disease. We must emphasize that any form of bodily introduction of these products into humans or animals is strictly prohibited by law. It is essential to adhere to these guidelines to ensure compliance with legal and ethical standards in research and experimentation.