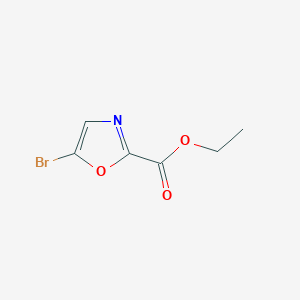
Ethyl 5-bromooxazole-2-carboxylate
- Click on QUICK INQUIRY to receive a quote from our team of experts.
- With the quality product at a COMPETITIVE price, you can focus more on your research.
Overview
Description
Ethyl 5-bromooxazole-2-carboxylate is a chemical compound with the molecular formula C6H6BrNO3 It is a derivative of oxazole, a five-membered aromatic heterocycle containing one oxygen and one nitrogen atom
Preparation Methods
Synthetic Routes and Reaction Conditions
Ethyl 5-bromooxazole-2-carboxylate can be synthesized through several methods. One common approach involves the bromination of ethyl oxazole-2-carboxylate. The reaction typically uses bromine or a brominating agent such as N-bromosuccinimide (NBS) in the presence of a catalyst like iron(III) bromide. The reaction is carried out under controlled conditions to ensure selective bromination at the 5-position of the oxazole ring.
Industrial Production Methods
In an industrial setting, the production of this compound may involve continuous flow processes to enhance efficiency and yield. The use of automated systems and optimized reaction conditions can help in scaling up the production while maintaining the purity and quality of the compound.
Chemical Reactions Analysis
Types of Reactions
Ethyl 5-bromooxazole-2-carboxylate undergoes various chemical reactions, including:
Substitution Reactions: The bromine atom at the 5-position can be substituted with other nucleophiles, such as amines or thiols, under appropriate conditions.
Oxidation and Reduction: The oxazole ring can participate in oxidation and reduction reactions, leading to the formation of different derivatives.
Coupling Reactions: The compound can undergo coupling reactions, such as Suzuki-Miyaura coupling, to form carbon-carbon bonds with other aromatic or aliphatic groups.
Common Reagents and Conditions
Nucleophilic Substitution: Reagents like sodium azide or potassium thiolate can be used in the presence of a base to facilitate substitution reactions.
Oxidation: Oxidizing agents such as potassium permanganate or hydrogen peroxide can be employed to oxidize the oxazole ring.
Coupling: Palladium catalysts and boronic acids are commonly used in coupling reactions.
Major Products Formed
The major products formed from these reactions depend on the specific reagents and conditions used. For example, nucleophilic substitution with an amine can yield an amino-substituted oxazole derivative, while coupling reactions can produce biaryl compounds.
Scientific Research Applications
Ethyl 5-bromooxazole-2-carboxylate has several applications in scientific research:
Medicinal Chemistry: It is used as a building block in the synthesis of pharmaceutical compounds, particularly those with potential anticancer, antimicrobial, and anti-inflammatory properties.
Organic Synthesis: The compound serves as an intermediate in the synthesis of more complex molecules, including natural products and heterocyclic compounds.
Material Science: It is used in the development of novel materials with specific electronic or optical properties.
Biological Studies: Researchers use this compound to study the biological activity of oxazole derivatives and their interactions with various biomolecules.
Mechanism of Action
The mechanism of action of ethyl 5-bromooxazole-2-carboxylate depends on its specific application. In medicinal chemistry, the compound may interact with molecular targets such as enzymes or receptors, modulating their activity. The bromine atom and the oxazole ring play crucial roles in these interactions, influencing the compound’s binding affinity and specificity.
Comparison with Similar Compounds
Ethyl 5-bromooxazole-2-carboxylate can be compared with other similar compounds, such as:
Ethyl 2-bromooxazole-5-carboxylate: This isomer has the bromine atom at the 2-position instead of the 5-position, leading to different reactivity and applications.
Ethyl 5-chlorooxazole-2-carboxylate: The chlorine atom in place of bromine affects the compound’s chemical properties and reactivity.
Ethyl oxazole-2-carboxylate: The absence of a halogen atom makes this compound less reactive in substitution reactions but still useful in organic synthesis.
Biological Activity
Ethyl 5-bromooxazole-2-carboxylate is a heterocyclic compound that has garnered interest in medicinal chemistry due to its potential biological activities, particularly in the fields of antimicrobial, anticancer, and anti-inflammatory research. This article explores the biological activity of this compound, its mechanisms of action, and relevant case studies.
Chemical Structure and Properties
This compound features an oxazole ring with a bromo substituent at the 5-position and an ethyl ester at the 2-position. Its chemical formula is C6H6BrNO3.
The biological activity of this compound is primarily attributed to its ability to interact with various biological targets, such as enzymes and receptors. The bromine atom and the oxazole ring are crucial for these interactions, influencing binding affinity and specificity. The compound may undergo several types of chemical reactions:
- Substitution Reactions: The bromine atom can be replaced by nucleophiles like amines or thiols.
- Oxidation and Reduction: The oxazole ring can participate in redox reactions.
- Coupling Reactions: It can form carbon-carbon bonds through coupling reactions like Suzuki-Miyaura coupling.
Antimicrobial Activity
Research indicates that this compound exhibits significant antimicrobial properties. In vitro studies have shown its effectiveness against various bacterial strains, including resistant strains.
Table 1: Antimicrobial Activity Data
Compound | Microbial Strain | Minimum Inhibitory Concentration (MIC) |
---|---|---|
This compound | Staphylococcus aureus | 15 µg/mL |
Escherichia coli | 20 µg/mL | |
Pseudomonas aeruginosa | 25 µg/mL |
The compound's ability to inhibit bacterial growth suggests potential applications in developing new antibiotics.
Anticancer Properties
This compound has also been studied for its anticancer effects. In a study involving cancer cell lines, it demonstrated cytotoxic effects, leading to apoptosis in cancer cells.
Case Study: Cytotoxicity Against Cancer Cell Lines
In a study conducted on various cancer cell lines (e.g., MCF-7 for breast cancer), this compound exhibited an IC50 value of approximately 30 µM, indicating a promising therapeutic index.
Structure-Activity Relationship (SAR)
The structure-activity relationship of this compound has been explored to understand how modifications affect its biological activity. Substitutions at different positions on the oxazole ring can enhance or diminish its efficacy against specific targets.
Table 2: SAR Analysis
Compound Variation | Biological Activity | Observations |
---|---|---|
This compound | High antimicrobial activity | Effective against resistant strains |
Ethyl 5-chlorooxazole-2-carboxylate | Moderate activity | Less effective than bromo variant |
Ethyl oxazole-2-carboxylate | Low activity | Lacks halogen substitution |
Properties
IUPAC Name |
ethyl 5-bromo-1,3-oxazole-2-carboxylate |
Source
|
---|---|---|
Details | Computed by LexiChem 2.6.6 (PubChem release 2019.06.18) | |
Source | PubChem | |
URL | https://pubchem.ncbi.nlm.nih.gov | |
Description | Data deposited in or computed by PubChem | |
InChI |
InChI=1S/C6H6BrNO3/c1-2-10-6(9)5-8-3-4(7)11-5/h3H,2H2,1H3 |
Source
|
Details | Computed by InChI 1.0.5 (PubChem release 2019.06.18) | |
Source | PubChem | |
URL | https://pubchem.ncbi.nlm.nih.gov | |
Description | Data deposited in or computed by PubChem | |
InChI Key |
XPNHEUUMAHKJLK-UHFFFAOYSA-N |
Source
|
Details | Computed by InChI 1.0.5 (PubChem release 2019.06.18) | |
Source | PubChem | |
URL | https://pubchem.ncbi.nlm.nih.gov | |
Description | Data deposited in or computed by PubChem | |
Canonical SMILES |
CCOC(=O)C1=NC=C(O1)Br |
Source
|
Details | Computed by OEChem 2.1.5 (PubChem release 2019.06.18) | |
Source | PubChem | |
URL | https://pubchem.ncbi.nlm.nih.gov | |
Description | Data deposited in or computed by PubChem | |
Molecular Formula |
C6H6BrNO3 |
Source
|
Details | Computed by PubChem 2.1 (PubChem release 2019.06.18) | |
Source | PubChem | |
URL | https://pubchem.ncbi.nlm.nih.gov | |
Description | Data deposited in or computed by PubChem | |
Molecular Weight |
220.02 g/mol |
Source
|
Details | Computed by PubChem 2.1 (PubChem release 2021.05.07) | |
Source | PubChem | |
URL | https://pubchem.ncbi.nlm.nih.gov | |
Description | Data deposited in or computed by PubChem | |
Disclaimer and Information on In-Vitro Research Products
Please be aware that all articles and product information presented on BenchChem are intended solely for informational purposes. The products available for purchase on BenchChem are specifically designed for in-vitro studies, which are conducted outside of living organisms. In-vitro studies, derived from the Latin term "in glass," involve experiments performed in controlled laboratory settings using cells or tissues. It is important to note that these products are not categorized as medicines or drugs, and they have not received approval from the FDA for the prevention, treatment, or cure of any medical condition, ailment, or disease. We must emphasize that any form of bodily introduction of these products into humans or animals is strictly prohibited by law. It is essential to adhere to these guidelines to ensure compliance with legal and ethical standards in research and experimentation.