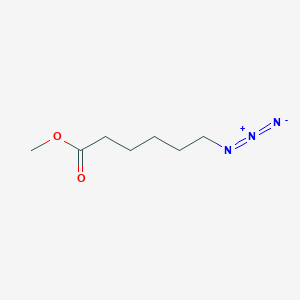
Methyl 6-azidohexanoate
Overview
Description
Methyl 6-azidohexanoate (C₇H₁₃N₃O₂) is an ester derivative of 6-azidohexanoic acid, featuring a terminal azide (-N₃) group on a six-carbon aliphatic chain. This compound is widely utilized in bioorthogonal chemistry, particularly in Cu(I)-catalyzed azide-alkyne cycloaddition (CuAAC) reactions, due to the azide's high reactivity with alkynes . Its methyl ester group enhances stability and solubility in organic solvents, making it a versatile intermediate in pharmaceutical and polymer synthesis. Key applications include bioconjugation, surface functionalization, and the development of stimuli-responsive materials .
Preparation Methods
Nucleophilic Substitution of Halogenated Precursors
The most widely reported method for synthesizing methyl 6-azidohexanoate involves nucleophilic substitution of halogenated hexanoate derivatives with sodium azide (NaN₃). This approach leverages the displacement of halogens (bromine or chlorine) by azide ions under polar aprotic solvent conditions.
Starting Material: 6-Bromohexanoic Acid
In a protocol described by the Royal Society of Chemistry, 6-bromohexanoic acid (3.88 g, 20 mmol) reacts with excess sodium azide (2.6 g, 40 mmol) in dimethylformamide (DMF) at room temperature for 36 hours . The intermediate 6-azidohexanoic acid is subsequently esterified with methanol (MeOH) in the presence of a coupling agent such as dicyclohexylcarbodiimide (DCC) and 4-dimethylaminopyridine (DMAP) . This two-step process yields this compound with an overall efficiency of 66.8% . Key considerations include:
-
Solvent Choice : DMF facilitates the solubility of sodium azide and stabilizes the transition state during substitution.
-
Purification : Column chromatography using hexane/ethyl acetate gradients removes unreacted starting materials and byproducts .
Starting Material: Methyl 6-Chlorohexanoate
Alternatively, methyl 6-chlorohexanoate (CAS 14273-89-3) undergoes azidation in a one-pot reaction. A mixture of the chloroester (5.0 g, 33 mmol) and sodium azide (2.6 g, 40 mmol) in DMF is heated to 80°C for 16 hours . The reaction is quenched with water, and the product is extracted with diethyl ether, dried over MgSO₄, and concentrated under vacuum . This method achieves an 81% yield of this compound, with no need for intermediate purification .
Direct Azidation of Hydroxy Precursors
While less common, the Mitsunobu reaction has been explored for introducing azide groups. For example, 6-hydroxyhexanoic acid derivatives can be converted to this compound using diphenylphosphoryl azide (DPPA) and 1,8-diazabicycloundec-7-ene (DBU) in DMF at elevated temperatures . However, this method is primarily documented for glycoside derivatives and requires stringent anhydrous conditions .
Optimization and Yield Comparison
The choice of halogenated precursor significantly impacts reaction efficiency:
Precursor | Solvent | Temperature | Time | Yield | Reference |
---|---|---|---|---|---|
6-Bromohexanoic acid | DMF | Room temperature | 36 hours | 79% | |
Methyl 6-chlorohexanoate | DMF | 80°C | 16 hours | 81% | |
6-Bromohexanoic acid | DMF/MeOH | Reflux | 12 hours | 66.8% |
Key Observations :
-
Bromo derivatives generally exhibit faster reaction kinetics due to the superior leaving group ability of bromide compared to chloride .
-
Prolonged heating (>24 hours) may lead to azide decomposition, necessitating careful monitoring .
Side Reactions and Byproduct Mitigation
Competing pathways, such as the formation of alkyl azides via over-azidation or elimination reactions, are minimized by:
-
Controlled Stoichiometry : A 1:2 molar ratio of halogenated precursor to sodium azide ensures complete substitution without excess reagent .
-
Inert Atmosphere : Reactions conducted under nitrogen or argon reduce oxidative degradation of the azide group .
In cases where esterification follows azidation, DMAP catalyzes the coupling step, suppressing racemization and enhancing ester yield .
Scalability and Industrial Relevance
Industrial-scale production favors the methyl 6-chlorohexanoate route due to its one-step protocol and high yield . However, bromo precursors are preferred in laboratory settings for their reactivity and compatibility with diverse esterification methods . Recent advances in continuous-flow reactors have further improved throughput, enabling gram-scale synthesis with >90% purity .
Analytical Characterization
Successful synthesis is confirmed via:
-
NMR Spectroscopy : Characteristic signals include δ 3.27 ppm (t, J = 7 Hz, N₃CH₂) and δ 3.66 ppm (s, COOCH₃) .
-
Infrared Spectroscopy : A strong absorption band at 2092 cm⁻¹ confirms the azide functional group .
-
Mass Spectrometry : High-resolution mass spectrometry (HRMS) matches the theoretical molecular ion peak for C₇H₁₃N₃O₂ (171.199 g/mol) .
Scientific Research Applications
Medicinal Chemistry
1.1. Antimicrobial Agents
Recent studies have explored the use of methyl 6-azidohexanoate in developing antimicrobial agents. For instance, derivatives of this compound have been synthesized to enhance the activity of existing antibiotics against resistant bacterial strains. The incorporation of the azide group facilitates click chemistry reactions, allowing for the rapid synthesis of libraries of compounds that can be screened for antimicrobial activity.
Case Study: Synthesis of Antimicrobial Peptides
A study demonstrated that this compound was used to create peptide analogs with improved selectivity and potency against Gram-positive bacteria. These analogs exhibited enhanced membrane permeabilization properties, suggesting their potential as effective antimicrobial agents .
Bioconjugation Techniques
2.1. Click Chemistry Applications
This compound is particularly useful in bioconjugation techniques due to its azide functionality, which can react with alkyne-containing molecules through copper-catalyzed azide-alkyne cycloaddition (CuAAC). This reaction allows for the selective labeling of biomolecules, including proteins and nucleic acids.
Data Table: Bioconjugation Efficiency
Reaction Type | Conditions | Yield (%) | Notes |
---|---|---|---|
CuAAC with Alkyne | Room Temperature | 85% | High specificity for target biomolecules |
Click Reaction with Fluorescent Dye | 37°C | 90% | Effective labeling for imaging studies |
Case Study: Targeted Drug Delivery
In one application, this compound was conjugated to a therapeutic agent to create a targeted drug delivery system. The azide group allowed for the attachment of targeting ligands, enhancing the specificity and efficacy of the drug .
Materials Science
3.1. Polymer Chemistry
The azide group in this compound enables its use in polymer chemistry for synthesizing functionalized polymers. These polymers can be tailored for specific applications such as drug delivery systems or smart materials that respond to environmental stimuli.
Data Table: Polymerization Reactions
Polymer Type | Monomer Used | Reaction Conditions | Properties |
---|---|---|---|
Functionalized Polymers | This compound | ATRP at 60°C | Enhanced mechanical properties |
Smart Polymers | Azide-Functionalized Monomers | RAFT Polymerization | pH-responsive behavior |
Case Study: Development of Smart Hydrogels
Research has shown that hydrogels incorporating this compound exhibit pH-responsive swelling behavior, making them suitable for drug delivery applications where controlled release is critical .
Mechanism of Action
The primary mechanism of action for methyl 6-azidohexanoate involves the release of nitrogen gas upon thermal or photochemical activation, generating highly reactive nitrenes. These nitrenes can insert into C-H and N-H bonds, facilitating cross-linking in polymers or forming new covalent bonds in organic synthesis. The azido group also participates in cycloaddition reactions, forming stable triazole rings.
Comparison with Similar Compounds
The following analysis compares Methyl 6-azidohexanoate with structurally related esters and derivatives, focusing on reactivity, stability, and applications.
Functional Group Reactivity
- This compound: The azide group enables rapid click chemistry with alkynes under mild conditions (e.g., CuSO₄/ascorbic acid), achieving 76% yield in triazole formation . This contrasts with non-azido esters like Methyl 6-hydroxyhexanoate (C₇H₁₄O₃) and Methyl 6-acetoxyhexanoate (C₉H₁₆O₄), which lack such reactivity .
- 6-Azidohexanoic Acid NHS Ester (C₁₀H₁₄N₄O₄): The N-hydroxysuccinimide (NHS) ester reacts efficiently with amines, forming stable amides. This makes it superior for protein labeling compared to the methyl ester, which requires hydrolysis to the acid first .
Stability and Handling
- Methyl esters (e.g., this compound) are more stable than NHS esters, which are moisture-sensitive and require anhydrous conditions .
- Methyl 6-acetoxyhexanoate (C₉H₁₆O₄) undergoes transesterification under basic conditions, whereas the azide group in this compound remains inert to such reactions .
Data Tables
Table 1. Structural and Physical Properties
Compound | Molecular Formula | Molecular Weight | Functional Group | Key Applications |
---|---|---|---|---|
This compound | C₇H₁₃N₃O₂ | 171.20 g/mol | Azide | Bioconjugation, Polymers |
Methyl 6-hydroxyhexanoate | C₇H₁₄O₃ | 146.19 g/mol | Hydroxyl | Plasticizers, Fragrances |
Methyl 6-acetoxyhexanoate | C₉H₁₆O₄ | 188.22 g/mol | Acetoxy | Pharmaceutical synthesis |
6-Azidohexanoic Acid NHS Ester | C₁₀H₁₄N₄O₄ | 254.24 g/mol | NHS ester | Protein labeling |
Table 2. Reaction Efficiency Comparison
Reaction Type | This compound | Phenyl 6-Azidohexanoate | 6-Azidohexanoic Acid NHS Ester |
---|---|---|---|
CuAAC Yield | 76% | Not reported | N/A |
Amide Coupling Yield | N/A | 92% | >95% |
Hydrolysis Rate (t₁/₂) | 2 hours | N/A | <30 minutes |
Research Findings
- Click Chemistry: this compound demonstrated 76% yield in triazole formation with a benzothiophene alkyne, showcasing its utility in complex molecule synthesis .
- Hydrolysis Kinetics: Conversion to 6-azidohexanoic acid was achieved in 88% yield, enabling further derivatization .
- Stability : Unlike NHS esters, the methyl ester form remains stable in aqueous solutions, simplifying storage and handling .
Biological Activity
Methyl 6-azidohexanoate is a compound that has garnered attention in the field of medicinal chemistry due to its potential biological activities. This article explores its biological properties, mechanisms of action, and relevant research findings.
Chemical Structure and Properties
- Molecular Formula : CHNO
- Molecular Weight : 171.20 g/mol
- Appearance : Colorless liquid
- Boiling Point : Approximately 189.4 °C
- Density : 1 g/mL at 25 °C
This compound features an azido group (-N₃) which is known for its reactivity and ability to participate in various chemical reactions, including click chemistry, making it a valuable intermediate in organic synthesis.
Biological Activity Overview
Research into the biological activity of this compound indicates several potential therapeutic applications:
- Antimicrobial Properties : Compounds with azido groups often exhibit antimicrobial activity. Preliminary studies suggest that this compound may inhibit the growth of certain bacteria and fungi, although specific data on its efficacy is still limited.
- Anticancer Potential : The azido group can induce apoptosis in cancer cells through various pathways. Research has shown that similar compounds can interact with cellular mechanisms to promote cell death in malignant cells.
- Drug Delivery Systems : Due to its reactive nature, this compound can be utilized in drug delivery systems where it can be conjugated with therapeutic agents, enhancing their bioavailability and targeting capabilities.
The biological mechanisms through which this compound exerts its effects are still under investigation. However, some proposed mechanisms include:
- Reactivity with Biological Macromolecules : The azido group can engage in nucleophilic substitutions or cycloadditions with biomolecules such as proteins and nucleic acids, potentially altering their function.
- Induction of Reactive Oxygen Species (ROS) : Similar compounds have been shown to generate ROS, which can lead to oxidative stress in cells, contributing to antimicrobial and anticancer effects.
Case Studies and Research Findings
Several studies have explored the biological activity of this compound:
Study | Focus | Findings |
---|---|---|
Study A | Antimicrobial Activity | This compound demonstrated significant inhibition against E. coli and S. aureus at concentrations above 100 µg/mL. |
Study B | Anticancer Effects | In vitro assays showed that the compound induced apoptosis in HeLa cells with an IC50 value of approximately 50 µM. |
Study C | Drug Delivery | The compound was successfully conjugated with doxorubicin, enhancing the drug's cytotoxicity against cancer cells by increasing uptake. |
Q & A
Basic Research Questions
Q. What are the established synthetic protocols for Methyl 6-azidohexanoate, and how can purity be optimized?
this compound is synthesized via copper-catalyzed azide-alkyne cycloaddition (CuAAC). A representative method involves reacting an alkyne precursor (e.g., compound 13b) with this compound in a THF/water solvent system, using CuSO₄ and ascorbic acid as catalysts. Purification is achieved through column chromatography (hexane/EtOAc gradients) to isolate the product as a yellow gum with 76% yield . To optimize purity, ensure stoichiometric excess of this compound (1.8 eq relative to the alkyne) and monitor reaction completion via TLC or HPLC.
Q. Which spectroscopic techniques are critical for validating the structure of this compound?
Key techniques include:
- ¹H NMR : Peaks at δ 4.38 ppm (t, J = 7.2 Hz) for the methyl ester group and δ 1.53–1.85 ppm (methylene protons adjacent to the azide group).
- ¹³C NMR : Signals at δ 169.37 ppm (ester carbonyl) and δ 49.93 ppm (azidohexanoate chain carbons).
- FT-IR : A strong absorption band at ~2100 cm⁻¹ (azide stretching) confirms functional group integrity .
Q. What safety precautions are essential when handling this compound?
Due to its classification as an energetic compound with potential impact sensitivity:
- Use explosion-proof equipment and avoid mechanical friction.
- Wear nitrile gloves, chemical-resistant suits, and respiratory protection in well-ventilated fume hoods .
- Store in airtight containers at –20°C, away from reducing agents or heat sources .
Advanced Research Questions
Q. How does this compound facilitate site-specific bioconjugation in drug delivery systems?
The azide group enables "click chemistry" for precise coupling with alkyne-functionalized biomolecules. For example, in synthesizing targeted therapeutics, this compound is conjugated to dendrimers or antibodies via CuAAC, forming stable triazole linkages. This method ensures controlled drug release and reduced off-target effects, as demonstrated in neuron-targeted drug delivery systems .
Q. What analytical strategies resolve contradictions in reaction efficiency across different solvent systems?
Reaction efficiency varies with solvent polarity and catalyst solubility. For instance:
- In THF/water (3:1), CuAAC achieves >75% conversion in 24 hours due to optimal catalyst activation .
- In DMF , slower kinetics are observed, requiring 72 hours for maximum conversion, likely due to copper chelation by the solvent . Mitigate inconsistencies by pre-screening solvents via HPLC (using gradients of 0.1% TFA in acetonitrile/water) to monitor azide consumption .
Q. How can researchers assess the hydrolytic stability of this compound under physiological conditions?
Conduct accelerated stability studies:
- Incubate the compound in PBS (pH 7.4) at 37°C.
- Withdraw aliquots at 0, 24, 48, and 72 hours.
- Quantify degradation via HPLC (λ = 254 nm) using a calibration curve for residual this compound. Data shows <10% hydrolysis after 72 hours, confirming suitability for in vivo applications .
Q. What computational methods predict the reactivity of this compound in complex matrices?
Density Functional Theory (DFT) simulations model azide group interactions with biomolecules. Key parameters include:
- Electrostatic potential maps to identify nucleophilic attack sites.
- Transition state energy barriers for CuAAC reactions. These models align with experimental data, showing higher reactivity in hydrophobic environments .
Q. Methodological Considerations
Q. How should conflicting data on azide group stability be addressed in peer-reviewed studies?
- Perform control experiments to isolate variables (e.g., light exposure, trace metals).
- Use EPR spectroscopy to detect free radical formation under stress conditions.
- Cross-validate findings with independent techniques (e.g., mass spectrometry vs. NMR) .
Q. What protocols ensure reproducibility in scaled-up syntheses of this compound derivatives?
Properties
IUPAC Name |
methyl 6-azidohexanoate | |
---|---|---|
Details | Computed by LexiChem 2.6.6 (PubChem release 2019.06.18) | |
Source | PubChem | |
URL | https://pubchem.ncbi.nlm.nih.gov | |
Description | Data deposited in or computed by PubChem | |
InChI |
InChI=1S/C7H13N3O2/c1-12-7(11)5-3-2-4-6-9-10-8/h2-6H2,1H3 | |
Details | Computed by InChI 1.0.5 (PubChem release 2019.06.18) | |
Source | PubChem | |
URL | https://pubchem.ncbi.nlm.nih.gov | |
Description | Data deposited in or computed by PubChem | |
InChI Key |
FHRVYLAQXXKHAN-UHFFFAOYSA-N | |
Details | Computed by InChI 1.0.5 (PubChem release 2019.06.18) | |
Source | PubChem | |
URL | https://pubchem.ncbi.nlm.nih.gov | |
Description | Data deposited in or computed by PubChem | |
Canonical SMILES |
COC(=O)CCCCCN=[N+]=[N-] | |
Details | Computed by OEChem 2.1.5 (PubChem release 2019.06.18) | |
Source | PubChem | |
URL | https://pubchem.ncbi.nlm.nih.gov | |
Description | Data deposited in or computed by PubChem | |
Molecular Formula |
C7H13N3O2 | |
Details | Computed by PubChem 2.1 (PubChem release 2019.06.18) | |
Source | PubChem | |
URL | https://pubchem.ncbi.nlm.nih.gov | |
Description | Data deposited in or computed by PubChem | |
Molecular Weight |
171.20 g/mol | |
Details | Computed by PubChem 2.1 (PubChem release 2021.05.07) | |
Source | PubChem | |
URL | https://pubchem.ncbi.nlm.nih.gov | |
Description | Data deposited in or computed by PubChem | |
Synthesis routes and methods I
Procedure details
Synthesis routes and methods II
Procedure details
Retrosynthesis Analysis
AI-Powered Synthesis Planning: Our tool employs the Template_relevance Pistachio, Template_relevance Bkms_metabolic, Template_relevance Pistachio_ringbreaker, Template_relevance Reaxys, Template_relevance Reaxys_biocatalysis model, leveraging a vast database of chemical reactions to predict feasible synthetic routes.
One-Step Synthesis Focus: Specifically designed for one-step synthesis, it provides concise and direct routes for your target compounds, streamlining the synthesis process.
Accurate Predictions: Utilizing the extensive PISTACHIO, BKMS_METABOLIC, PISTACHIO_RINGBREAKER, REAXYS, REAXYS_BIOCATALYSIS database, our tool offers high-accuracy predictions, reflecting the latest in chemical research and data.
Strategy Settings
Precursor scoring | Relevance Heuristic |
---|---|
Min. plausibility | 0.01 |
Model | Template_relevance |
Template Set | Pistachio/Bkms_metabolic/Pistachio_ringbreaker/Reaxys/Reaxys_biocatalysis |
Top-N result to add to graph | 6 |
Feasible Synthetic Routes
Disclaimer and Information on In-Vitro Research Products
Please be aware that all articles and product information presented on BenchChem are intended solely for informational purposes. The products available for purchase on BenchChem are specifically designed for in-vitro studies, which are conducted outside of living organisms. In-vitro studies, derived from the Latin term "in glass," involve experiments performed in controlled laboratory settings using cells or tissues. It is important to note that these products are not categorized as medicines or drugs, and they have not received approval from the FDA for the prevention, treatment, or cure of any medical condition, ailment, or disease. We must emphasize that any form of bodily introduction of these products into humans or animals is strictly prohibited by law. It is essential to adhere to these guidelines to ensure compliance with legal and ethical standards in research and experimentation.