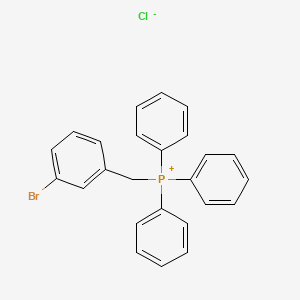
(3-BroMobenzyl)triphenylphosphoniuM chloride
Overview
Description
(3-BroMobenzyl)triphenylphosphoniuM chloride: is an organophosphorus compound with the molecular formula C25H21BrClP and a molecular weight of 467.77 g/mol . This compound is characterized by the presence of a triphenylphosphonium group attached to a 3-bromobenzyl moiety. It is commonly used in organic synthesis and has various applications in scientific research.
Preparation Methods
Synthetic Routes and Reaction Conditions: The synthesis of (3-BroMobenzyl)triphenylphosphoniuM chloride typically involves the reaction of triphenylphosphine with 3-bromobenzyl chloride. The reaction is usually carried out in an organic solvent such as toluene or xylene under reflux conditions. The product is then purified by recrystallization or column chromatography to obtain a high-purity compound .
Industrial Production Methods: In industrial settings, the synthesis of this compound can be scaled up using similar reaction conditions. The use of microwave irradiation has been reported to accelerate the reaction, resulting in higher yields and shorter reaction times . This method involves the microwave-assisted reaction of triphenylphosphine with 3-bromobenzyl chloride in the absence of a solvent or in the presence of a minimal amount of solvent.
Chemical Reactions Analysis
Types of Reactions: (3-BroMobenzyl)triphenylphosphoniuM chloride undergoes various types of chemical reactions, including:
Substitution Reactions: The bromine atom in the 3-bromobenzyl group can be substituted with other nucleophiles, such as azides or amines.
Oxidation and Reduction Reactions: The compound can participate in oxidation and reduction reactions, often involving the triphenylphosphonium group.
Cyclization Reactions: It can undergo cyclization reactions to form cyclic compounds.
Common Reagents and Conditions:
Substitution Reactions: Common reagents include sodium azide, primary amines, and other nucleophiles.
Oxidation and Reduction Reactions: Reagents such as hydrogen peroxide or sodium borohydride are used under mild conditions.
Cyclization Reactions: These reactions often require the presence of a base, such as potassium carbonate, and are conducted under reflux conditions.
Major Products Formed:
Substitution Reactions: Products include azido derivatives, amine derivatives, and other substituted benzyltriphenylphosphonium compounds.
Oxidation and Reduction Reactions: Products include oxidized or reduced forms of the original compound.
Cyclization Reactions: Cyclic phosphonium salts are formed as major products.
Scientific Research Applications
Chemistry: (3-BroMobenzyl)triphenylphosphoniuM chloride is widely used as a reagent in organic synthesis. It serves as a precursor for the synthesis of various phosphonium salts and is employed in Wittig reactions to form alkenes .
Biology and Medicine: In biological research, this compound is used to study mitochondrial function due to its ability to target mitochondria. It has been investigated for its potential as a mitochondrial-targeting agent in cancer therapy .
Industry: In industrial applications, this compound is used in the production of polymers and as a catalyst in various chemical reactions .
Mechanism of Action
The mechanism of action of (3-BroMobenzyl)triphenylphosphoniuM chloride involves its ability to target and accumulate in mitochondria. The triphenylphosphonium group facilitates the compound’s entry into the mitochondria, where it can exert its effects. This targeting ability is due to the positive charge on the phosphonium group, which is attracted to the negatively charged mitochondrial membrane . Once inside the mitochondria, the compound can interfere with mitochondrial function, leading to apoptosis in cancer cells or other biological effects.
Comparison with Similar Compounds
- (3-Bromopropyl)triphenylphosphonium bromide
- (4-Bromobutyl)triphenylphosphonium bromide
- (6-Bromohexyl)triphenylphosphonium bromide
Comparison: (3-BroMobenzyl)triphenylphosphoniuM chloride is unique due to the presence of the 3-bromobenzyl group, which imparts distinct reactivity and targeting properties compared to other similar compounds. For example, (3-Bromopropyl)triphenylphosphonium bromide has a shorter alkyl chain, which affects its reactivity and biological activity . Similarly, (4-Bromobutyl)triphenylphosphonium bromide and (6-Bromohexyl)triphenylphosphonium bromide have different chain lengths, influencing their chemical and biological properties .
Properties
IUPAC Name |
(3-bromophenyl)methyl-triphenylphosphanium;chloride | |
---|---|---|
Details | Computed by Lexichem TK 2.7.0 (PubChem release 2021.05.07) | |
Source | PubChem | |
URL | https://pubchem.ncbi.nlm.nih.gov | |
Description | Data deposited in or computed by PubChem | |
InChI |
InChI=1S/C25H21BrP.ClH/c26-22-12-10-11-21(19-22)20-27(23-13-4-1-5-14-23,24-15-6-2-7-16-24)25-17-8-3-9-18-25;/h1-19H,20H2;1H/q+1;/p-1 | |
Details | Computed by InChI 1.0.6 (PubChem release 2021.05.07) | |
Source | PubChem | |
URL | https://pubchem.ncbi.nlm.nih.gov | |
Description | Data deposited in or computed by PubChem | |
InChI Key |
JQXLRBIBLGYXIN-UHFFFAOYSA-M | |
Details | Computed by InChI 1.0.6 (PubChem release 2021.05.07) | |
Source | PubChem | |
URL | https://pubchem.ncbi.nlm.nih.gov | |
Description | Data deposited in or computed by PubChem | |
Canonical SMILES |
C1=CC=C(C=C1)[P+](CC2=CC(=CC=C2)Br)(C3=CC=CC=C3)C4=CC=CC=C4.[Cl-] | |
Details | Computed by OEChem 2.3.0 (PubChem release 2021.05.07) | |
Source | PubChem | |
URL | https://pubchem.ncbi.nlm.nih.gov | |
Description | Data deposited in or computed by PubChem | |
Molecular Formula |
C25H21BrClP | |
Details | Computed by PubChem 2.1 (PubChem release 2021.05.07) | |
Source | PubChem | |
URL | https://pubchem.ncbi.nlm.nih.gov | |
Description | Data deposited in or computed by PubChem | |
Molecular Weight |
467.8 g/mol | |
Details | Computed by PubChem 2.1 (PubChem release 2021.05.07) | |
Source | PubChem | |
URL | https://pubchem.ncbi.nlm.nih.gov | |
Description | Data deposited in or computed by PubChem | |
Synthesis routes and methods
Procedure details
Disclaimer and Information on In-Vitro Research Products
Please be aware that all articles and product information presented on BenchChem are intended solely for informational purposes. The products available for purchase on BenchChem are specifically designed for in-vitro studies, which are conducted outside of living organisms. In-vitro studies, derived from the Latin term "in glass," involve experiments performed in controlled laboratory settings using cells or tissues. It is important to note that these products are not categorized as medicines or drugs, and they have not received approval from the FDA for the prevention, treatment, or cure of any medical condition, ailment, or disease. We must emphasize that any form of bodily introduction of these products into humans or animals is strictly prohibited by law. It is essential to adhere to these guidelines to ensure compliance with legal and ethical standards in research and experimentation.