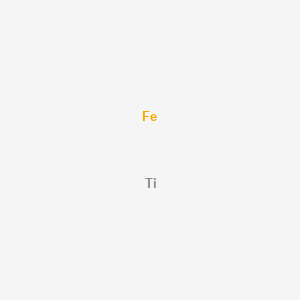
Iron, compound with titanium (1:1)
- Click on QUICK INQUIRY to receive a quote from our team of experts.
- With the quality product at a COMPETITIVE price, you can focus more on your research.
Overview
Description
Iron titanium compounds, such as iron titanate (Fe₂TiO₅), are fascinating materials known for their unique properties and wide-ranging applications. These compounds belong to the family of titanates and are characterized by the interaction of iron (Fe) and titanium (Ti) elements with oxygen (O). Iron titanium compounds are utilized in various fields due to their exceptional chemical and physical properties.
Preparation Methods
Synthetic Routes and Reaction Conditions
Iron titanium compounds can be synthesized through various methods, including solid-state reactions, sol-gel processes, and hydrothermal synthesis. One common method involves the solid-state reaction of iron oxide (Fe₂O₃) and titanium dioxide (TiO₂) at high temperatures. The reaction typically occurs at temperatures ranging from 800°C to 1200°C, resulting in the formation of iron titanate.
Industrial Production Methods
In industrial settings, the production of iron titanium compounds often involves the use of ilmenite (FeTiO₃) as a raw material. The ilmenite is subjected to a series of chemical processes, including reduction and oxidation, to extract titanium and iron oxides. These oxides are then combined and heated to produce iron titanium compounds. The Kroll process, which involves the reduction of titanium tetrachloride (TiCl₄) with magnesium, is also used to produce titanium metal, which can be further processed to form iron titanium compounds .
Chemical Reactions Analysis
Types of Reactions
Iron titanium compounds undergo various chemical reactions, including oxidation, reduction, and substitution reactions. These reactions are influenced by the presence of different reagents and reaction conditions.
Common Reagents and Conditions
Oxidation: Iron titanium compounds can be oxidized using oxygen or air at elevated temperatures. This process results in the formation of higher oxidation states of iron and titanium.
Reduction: Reduction reactions can be carried out using reducing agents such as hydrogen gas (H₂) or carbon monoxide (CO). These reactions typically occur at high temperatures and result in the formation of lower oxidation states of iron and titanium.
Substitution: Substitution reactions involve the replacement of one or more atoms in the compound with other atoms or groups. These reactions can be facilitated by the use of specific reagents and catalysts.
Major Products Formed
The major products formed from these reactions depend on the specific reaction conditions and reagents used. For example, the oxidation of iron titanium compounds can produce iron(III) oxide (Fe₂O₃) and titanium dioxide (TiO₂), while reduction reactions can yield iron(II) oxide (FeO) and titanium(III) oxide (Ti₂O₃) .
Scientific Research Applications
Iron titanium compounds have a wide range of scientific research applications due to their unique properties. Some of the key applications include:
Chemistry: Iron titanium compounds are used as catalysts in various chemical reactions, including photocatalysis and redox reactions.
Biology: These compounds are explored for their potential use in biological applications, such as antimicrobial agents and drug delivery systems.
Industry: In industrial applications, iron titanium compounds are used in the production of high-strength alloys, coatings, and electronic devices.
Mechanism of Action
The mechanism of action of iron titanium compounds involves their interaction with molecular targets and pathways. For example, in biological systems, iron titanium compounds can form hydrogen bonds with the constituents of microorganism cell-active sites, leading to the disruption of cell walls and membranes. This results in increased cell permeability and eventual cell death . In photocatalytic applications, the compounds can generate reactive oxygen species (ROS) that facilitate the degradation of organic pollutants .
Comparison with Similar Compounds
Iron titanium compounds can be compared with other similar compounds, such as iron aluminides, titanium aluminides, and nickel aluminides. These compounds share some common properties, such as high strength and corrosion resistance, but also exhibit unique characteristics:
Iron Aluminides: Known for their excellent oxidation resistance and high-temperature stability, iron aluminides are used in aerospace and automotive applications.
Titanium Aluminides: These compounds are lightweight and possess high strength-to-weight ratios, making them ideal for use in aircraft engines and other high-performance applications.
Iron titanium compounds stand out due to their unique combination of magnetic, electrical, and catalytic properties, which make them versatile materials for a wide range of applications.
Properties
CAS No. |
12023-04-0 |
---|---|
Molecular Formula |
FeTi |
Molecular Weight |
103.71 g/mol |
IUPAC Name |
iron;titanium |
InChI |
InChI=1S/Fe.Ti |
InChI Key |
IXQWNVPHFNLUGD-UHFFFAOYSA-N |
SMILES |
[Ti].[Fe] |
Canonical SMILES |
[Ti].[Fe] |
Key on ui other cas no. |
12023-04-0 |
Origin of Product |
United States |
Disclaimer and Information on In-Vitro Research Products
Please be aware that all articles and product information presented on BenchChem are intended solely for informational purposes. The products available for purchase on BenchChem are specifically designed for in-vitro studies, which are conducted outside of living organisms. In-vitro studies, derived from the Latin term "in glass," involve experiments performed in controlled laboratory settings using cells or tissues. It is important to note that these products are not categorized as medicines or drugs, and they have not received approval from the FDA for the prevention, treatment, or cure of any medical condition, ailment, or disease. We must emphasize that any form of bodily introduction of these products into humans or animals is strictly prohibited by law. It is essential to adhere to these guidelines to ensure compliance with legal and ethical standards in research and experimentation.