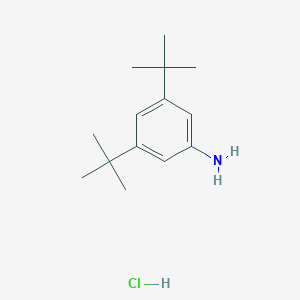
3,5-DI-Tert-butylaniline hydrochloride
- Click on QUICK INQUIRY to receive a quote from our team of experts.
- With the quality product at a COMPETITIVE price, you can focus more on your research.
Overview
Description
3,5-DI-Tert-butylaniline hydrochloride is an organic compound with the molecular formula C14H23N·HCl. It is a derivative of aniline, where the hydrogen atoms at the 3 and 5 positions of the benzene ring are replaced by tert-butyl groups. This compound is typically found as a white crystalline powder and is used in various chemical synthesis processes.
Preparation Methods
Synthetic Routes and Reaction Conditions: The synthesis of 3,5-DI-Tert-butylaniline hydrochloride generally involves the alkylation of aniline. One common method is the Friedel-Crafts alkylation, where aniline reacts with tert-butyl chloride in the presence of a Lewis acid catalyst such as aluminum chloride (AlCl3). The reaction proceeds as follows:
C6H5NH2+2(CH3)3CClAlCl3C6H3(C(CH3)3)2NH2
The resulting 3,5-DI-Tert-butylaniline is then converted to its hydrochloride salt by treatment with hydrochloric acid (HCl).
Industrial Production Methods: In industrial settings, the production of this compound may involve continuous flow processes to ensure higher efficiency and yield. The use of automated reactors and precise control of reaction conditions (temperature, pressure, and reactant concentrations) are crucial for large-scale synthesis.
Types of Reactions:
Oxidation: this compound can undergo oxidation reactions to form corresponding quinones or nitroso compounds. Common oxidizing agents include potassium permanganate (KMnO4) and hydrogen peroxide (H2O2).
Reduction: This compound can be reduced to form various amine derivatives. Reducing agents such as lithium aluminum hydride (LiAlH4) or sodium borohydride (NaBH4) are typically used.
Substitution: Electrophilic substitution reactions can occur on the aromatic ring, particularly at the para position relative to the amino group. Halogenation, nitration, and sulfonation are common substitution reactions.
Common Reagents and Conditions:
Oxidation: KMnO4 in acidic or neutral medium.
Reduction: LiAlH4 in dry ether or NaBH4 in methanol.
Substitution: Halogenation using bromine (Br2) in the presence of a catalyst like iron (Fe).
Major Products Formed:
Oxidation: Quinones, nitroso compounds.
Reduction: Secondary or tertiary amines.
Substitution: Halogenated, nitrated, or sulfonated derivatives.
Scientific Research Applications
3,5-DI-Tert-butylaniline hydrochloride is utilized in various fields of scientific research:
Chemistry: It serves as an intermediate in the synthesis of dyes, pigments, and other organic compounds.
Biology: It is used in the study of enzyme inhibition and protein interactions due to its structural similarity to certain biological molecules.
Medicine: Research into its potential as a pharmaceutical intermediate for the development of new drugs.
Industry: Employed in the manufacture of polymers and resins, where it acts as a stabilizer or modifier.
Mechanism of Action
The mechanism by which 3,5-DI-Tert-butylaniline hydrochloride exerts its effects depends on its application. In chemical reactions, its bulky tert-butyl groups can influence the steric and electronic properties of the molecule, affecting reactivity and selectivity. In biological systems, it may interact with enzymes or receptors, altering their activity through competitive inhibition or allosteric modulation.
Comparison with Similar Compounds
Aniline: The parent compound, lacking the tert-butyl groups.
2,4-DI-Tert-butylaniline: Similar structure but with tert-butyl groups at the 2 and 4 positions.
3,5-DI-Tert-butylphenol: Similar substitution pattern but with a hydroxyl group instead of an amino group.
Uniqueness: 3,5-DI-Tert-butylaniline hydrochloride is unique due to the specific positioning of the tert-butyl groups, which significantly impacts its chemical reactivity and physical properties. This makes it particularly useful in applications requiring steric hindrance or specific electronic effects.
Properties
IUPAC Name |
3,5-ditert-butylaniline;hydrochloride |
Source
|
---|---|---|
Details | Computed by LexiChem 2.6.6 (PubChem release 2019.06.18) | |
Source | PubChem | |
URL | https://pubchem.ncbi.nlm.nih.gov | |
Description | Data deposited in or computed by PubChem | |
InChI |
InChI=1S/C14H23N.ClH/c1-13(2,3)10-7-11(14(4,5)6)9-12(15)8-10;/h7-9H,15H2,1-6H3;1H |
Source
|
Details | Computed by InChI 1.0.5 (PubChem release 2019.06.18) | |
Source | PubChem | |
URL | https://pubchem.ncbi.nlm.nih.gov | |
Description | Data deposited in or computed by PubChem | |
InChI Key |
ISAHBRRSWDQIOE-UHFFFAOYSA-N |
Source
|
Details | Computed by InChI 1.0.5 (PubChem release 2019.06.18) | |
Source | PubChem | |
URL | https://pubchem.ncbi.nlm.nih.gov | |
Description | Data deposited in or computed by PubChem | |
Canonical SMILES |
CC(C)(C)C1=CC(=CC(=C1)N)C(C)(C)C.Cl |
Source
|
Details | Computed by OEChem 2.1.5 (PubChem release 2019.06.18) | |
Source | PubChem | |
URL | https://pubchem.ncbi.nlm.nih.gov | |
Description | Data deposited in or computed by PubChem | |
Molecular Formula |
C14H24ClN |
Source
|
Details | Computed by PubChem 2.1 (PubChem release 2019.06.18) | |
Source | PubChem | |
URL | https://pubchem.ncbi.nlm.nih.gov | |
Description | Data deposited in or computed by PubChem | |
Molecular Weight |
241.80 g/mol |
Source
|
Details | Computed by PubChem 2.1 (PubChem release 2021.05.07) | |
Source | PubChem | |
URL | https://pubchem.ncbi.nlm.nih.gov | |
Description | Data deposited in or computed by PubChem | |
Disclaimer and Information on In-Vitro Research Products
Please be aware that all articles and product information presented on BenchChem are intended solely for informational purposes. The products available for purchase on BenchChem are specifically designed for in-vitro studies, which are conducted outside of living organisms. In-vitro studies, derived from the Latin term "in glass," involve experiments performed in controlled laboratory settings using cells or tissues. It is important to note that these products are not categorized as medicines or drugs, and they have not received approval from the FDA for the prevention, treatment, or cure of any medical condition, ailment, or disease. We must emphasize that any form of bodily introduction of these products into humans or animals is strictly prohibited by law. It is essential to adhere to these guidelines to ensure compliance with legal and ethical standards in research and experimentation.