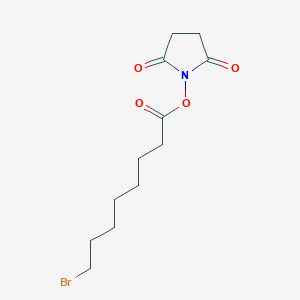
(2,5-dioxopyrrolidin-1-yl) 8-bromooctanoate
- Click on QUICK INQUIRY to receive a quote from our team of experts.
- With the quality product at a COMPETITIVE price, you can focus more on your research.
Overview
Description
(2,5-dioxopyrrolidin-1-yl) 8-bromooctanoate is a chemical compound with the molecular formula C12H18BrNO4 and a molecular weight of 320.2 g/mol. It is commonly used in various chemical and biological applications due to its unique properties and reactivity.
Preparation Methods
Synthetic Routes and Reaction Conditions
The synthesis of (2,5-dioxopyrrolidin-1-yl) 8-bromooctanoate typically involves the reaction of 8-bromo-octanoic acid with N-hydroxysuccinimide (NHS) in the presence of a coupling agent such as dicyclohexylcarbodiimide (DCC). The reaction is carried out in an organic solvent like methylene chloride under nitrogen atmosphere . The resulting product is then purified through recrystallization or chromatography.
Industrial Production Methods
Industrial production of this compound follows similar synthetic routes but on a larger scale. The process involves the use of high-purity reagents and controlled reaction conditions to ensure the consistency and quality of the final product. The compound is often produced in bulk and stored under specific conditions to maintain its stability.
Chemical Reactions Analysis
Types of Reactions
(2,5-dioxopyrrolidin-1-yl) 8-bromooctanoate undergoes various chemical reactions, including:
Substitution Reactions: The bromine atom can be substituted with other nucleophiles, leading to the formation of different derivatives.
Hydrolysis: The ester bond can be hydrolyzed to yield 8-bromo-octanoic acid and N-hydroxysuccinimide.
Common Reagents and Conditions
Nucleophiles: Common nucleophiles used in substitution reactions include amines, thiols, and alcohols.
Hydrolysis Conditions: Hydrolysis typically occurs under acidic or basic conditions, often using water or aqueous solutions.
Major Products
Substitution Products: Depending on the nucleophile used, various substituted derivatives of 8-bromo-octanoic acid can be formed.
Hydrolysis Products: The primary products of hydrolysis are 8-bromo-octanoic acid and N-hydroxysuccinimide.
Scientific Research Applications
(2,5-dioxopyrrolidin-1-yl) 8-bromooctanoate is widely used in scientific research due to its versatility:
Chemistry: It is used as a reagent in organic synthesis, particularly in the preparation of various derivatives and intermediates.
Biology: The compound is employed in biochemical studies, including enzyme inhibition and protein modification.
Industry: The compound is used in the production of specialty chemicals and materials.
Mechanism of Action
The mechanism of action of (2,5-dioxopyrrolidin-1-yl) 8-bromooctanoate involves its reactivity with nucleophiles. The bromine atom serves as a leaving group, allowing the compound to undergo substitution reactions. The ester bond can also be hydrolyzed, releasing 8-bromo-octanoic acid and N-hydroxysuccinimide . These reactions are facilitated by the presence of specific reagents and conditions.
Comparison with Similar Compounds
Similar Compounds
8-Bromooctanoic acid:
2,5-Dioxopyrrolidin-1-yl octanoate: Another ester derivative with comparable properties and applications.
Uniqueness
(2,5-dioxopyrrolidin-1-yl) 8-bromooctanoate is unique due to its combination of a bromine atom and an ester functional group, which provides distinct reactivity and versatility in various chemical and biological applications.
Properties
IUPAC Name |
(2,5-dioxopyrrolidin-1-yl) 8-bromooctanoate |
Source
|
---|---|---|
Details | Computed by LexiChem 2.6.6 (PubChem release 2019.06.18) | |
Source | PubChem | |
URL | https://pubchem.ncbi.nlm.nih.gov | |
Description | Data deposited in or computed by PubChem | |
InChI |
InChI=1S/C12H18BrNO4/c13-9-5-3-1-2-4-6-12(17)18-14-10(15)7-8-11(14)16/h1-9H2 |
Source
|
Details | Computed by InChI 1.0.5 (PubChem release 2019.06.18) | |
Source | PubChem | |
URL | https://pubchem.ncbi.nlm.nih.gov | |
Description | Data deposited in or computed by PubChem | |
InChI Key |
LHKDWGLZMFQSGB-UHFFFAOYSA-N |
Source
|
Details | Computed by InChI 1.0.5 (PubChem release 2019.06.18) | |
Source | PubChem | |
URL | https://pubchem.ncbi.nlm.nih.gov | |
Description | Data deposited in or computed by PubChem | |
Canonical SMILES |
C1CC(=O)N(C1=O)OC(=O)CCCCCCCBr |
Source
|
Details | Computed by OEChem 2.1.5 (PubChem release 2019.06.18) | |
Source | PubChem | |
URL | https://pubchem.ncbi.nlm.nih.gov | |
Description | Data deposited in or computed by PubChem | |
Molecular Formula |
C12H18BrNO4 |
Source
|
Details | Computed by PubChem 2.1 (PubChem release 2019.06.18) | |
Source | PubChem | |
URL | https://pubchem.ncbi.nlm.nih.gov | |
Description | Data deposited in or computed by PubChem | |
Molecular Weight |
320.18 g/mol |
Source
|
Details | Computed by PubChem 2.1 (PubChem release 2021.05.07) | |
Source | PubChem | |
URL | https://pubchem.ncbi.nlm.nih.gov | |
Description | Data deposited in or computed by PubChem | |
Disclaimer and Information on In-Vitro Research Products
Please be aware that all articles and product information presented on BenchChem are intended solely for informational purposes. The products available for purchase on BenchChem are specifically designed for in-vitro studies, which are conducted outside of living organisms. In-vitro studies, derived from the Latin term "in glass," involve experiments performed in controlled laboratory settings using cells or tissues. It is important to note that these products are not categorized as medicines or drugs, and they have not received approval from the FDA for the prevention, treatment, or cure of any medical condition, ailment, or disease. We must emphasize that any form of bodily introduction of these products into humans or animals is strictly prohibited by law. It is essential to adhere to these guidelines to ensure compliance with legal and ethical standards in research and experimentation.