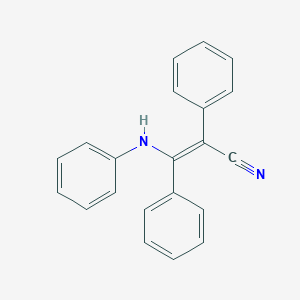
2,3-Diphenyl-3-phenylamino-acrylonitrile
- Click on QUICK INQUIRY to receive a quote from our team of experts.
- With the quality product at a COMPETITIVE price, you can focus more on your research.
Overview
Description
The compound identified as “2,3-Diphenyl-3-phenylamino-acrylonitrile” is a strong cation exchanger with increased potential for nonpolar interactions. It is commonly used in solid-phase extraction (SPE) applications due to its unique properties, which make it suitable for compounds with both cationic and non-polar characteristics .
Preparation Methods
Synthetic Routes and Reaction Conditions
The preparation of this compound involves the synthesis of a strong cation exchanger with a very low pKa. The presence of a benzene ring in the functional group increases the potential for nonpolar interactions, which is particularly important when conducting ion exchange from aqueous systems .
Industrial Production Methods
In industrial settings, the compound is produced in large quantities using scaled-up SPE cartridges. The typical matrices for this compound include aqueous samples, biological fluids, and buffered organics .
Chemical Reactions Analysis
Types of Reactions
The compound undergoes various types of reactions, including ion exchange reactions. These reactions are facilitated by the strong cation exchange properties of the compound, which allow it to interact with both cationic and non-polar compounds .
Common Reagents and Conditions
Common reagents used in these reactions include aqueous solutions and buffered organics. The conditions for these reactions typically involve maintaining a low pH to ensure the cation exchange properties are maximized .
Major Products Formed
The major products formed from these reactions are typically the purified compounds that have been separated from the mixture using the strong cation exchange properties of the compound .
Scientific Research Applications
The compound has a wide range of scientific research applications, including:
Mechanism of Action
The mechanism of action of this compound involves its strong cation exchange properties. It interacts with cationic compounds through ion exchange, while its nonpolar characteristics allow it to interact with nonpolar compounds. This dual functionality makes it highly effective in separating and purifying compounds from complex mixtures .
Comparison with Similar Compounds
Similar Compounds
Bond Elut PRS: Similar in pKa but lacks the benzene ring, resulting in lower nonpolar interactions.
Bond Elut SCX: Another strong cation exchanger with similar properties but different structural characteristics.
Uniqueness
The uniqueness of “2,3-Diphenyl-3-phenylamino-acrylonitrile” lies in its combination of strong cation exchange properties and increased potential for nonpolar interactions due to the presence of a benzene ring in its functional group. This makes it particularly effective in applications where both cationic and non-polar characteristics are important .
Properties
IUPAC Name |
(E)-3-anilino-2,3-diphenylprop-2-enenitrile |
Source
|
---|---|---|
Details | Computed by LexiChem 2.6.6 (PubChem release 2019.06.18) | |
Source | PubChem | |
URL | https://pubchem.ncbi.nlm.nih.gov | |
Description | Data deposited in or computed by PubChem | |
InChI |
InChI=1S/C21H16N2/c22-16-20(17-10-4-1-5-11-17)21(18-12-6-2-7-13-18)23-19-14-8-3-9-15-19/h1-15,23H/b21-20- |
Source
|
Details | Computed by InChI 1.0.5 (PubChem release 2019.06.18) | |
Source | PubChem | |
URL | https://pubchem.ncbi.nlm.nih.gov | |
Description | Data deposited in or computed by PubChem | |
InChI Key |
UOYMJQXYILXPDC-MRCUWXFGSA-N |
Source
|
Details | Computed by InChI 1.0.5 (PubChem release 2019.06.18) | |
Source | PubChem | |
URL | https://pubchem.ncbi.nlm.nih.gov | |
Description | Data deposited in or computed by PubChem | |
Canonical SMILES |
C1=CC=C(C=C1)C(=C(C2=CC=CC=C2)NC3=CC=CC=C3)C#N |
Source
|
Details | Computed by OEChem 2.1.5 (PubChem release 2019.06.18) | |
Source | PubChem | |
URL | https://pubchem.ncbi.nlm.nih.gov | |
Description | Data deposited in or computed by PubChem | |
Isomeric SMILES |
C1=CC=C(C=C1)/C(=C(/C2=CC=CC=C2)\NC3=CC=CC=C3)/C#N |
Source
|
Details | Computed by OEChem 2.1.5 (PubChem release 2019.06.18) | |
Source | PubChem | |
URL | https://pubchem.ncbi.nlm.nih.gov | |
Description | Data deposited in or computed by PubChem | |
Molecular Formula |
C21H16N2 |
Source
|
Details | Computed by PubChem 2.1 (PubChem release 2019.06.18) | |
Source | PubChem | |
URL | https://pubchem.ncbi.nlm.nih.gov | |
Description | Data deposited in or computed by PubChem | |
Molecular Weight |
296.4 g/mol |
Source
|
Details | Computed by PubChem 2.1 (PubChem release 2021.05.07) | |
Source | PubChem | |
URL | https://pubchem.ncbi.nlm.nih.gov | |
Description | Data deposited in or computed by PubChem | |
Disclaimer and Information on In-Vitro Research Products
Please be aware that all articles and product information presented on BenchChem are intended solely for informational purposes. The products available for purchase on BenchChem are specifically designed for in-vitro studies, which are conducted outside of living organisms. In-vitro studies, derived from the Latin term "in glass," involve experiments performed in controlled laboratory settings using cells or tissues. It is important to note that these products are not categorized as medicines or drugs, and they have not received approval from the FDA for the prevention, treatment, or cure of any medical condition, ailment, or disease. We must emphasize that any form of bodily introduction of these products into humans or animals is strictly prohibited by law. It is essential to adhere to these guidelines to ensure compliance with legal and ethical standards in research and experimentation.