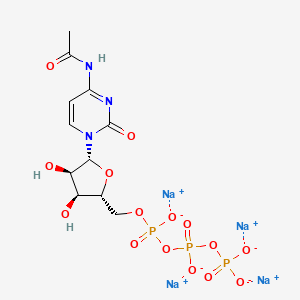
N4-Acetylcytidine triphosphate (sodium)
Overview
Description
N4-Acetylcytidine triphosphate (sodium) (ac4CTP sodium) is a chemically modified nucleotide analog with the molecular formula C₁₁H₁₄N₃Na₄O₁₅P₃ and a molecular weight of 613.12 . It serves as a substrate for T7 RNA polymerase in in vitro transcription systems, enabling the incorporation of N4-acetylcytidine into RNA strands . This modification enhances RNA stability and functionality in experimental settings. The compound exhibits high solubility in water (≥125 mg/mL) and is stored at -80°C under nitrogen protection to maintain stability . Its primary applications include studying RNA modification mechanisms and synthesizing modified RNA for therapeutic or diagnostic research.
Preparation Methods
Synthetic Routes and Reaction Conditions
The synthesis of N4-Acetylcytidine triphosphate (sodium) typically involves the acetylation of cytidine triphosphate. The process begins with the protection of the hydroxyl groups of cytidine triphosphate, followed by the selective acetylation of the amino group at the N4 position. The reaction is usually carried out in the presence of acetic anhydride and a base such as pyridine. After acetylation, the protecting groups are removed to yield N4-Acetylcytidine triphosphate. The final product is then converted to its sodium salt form for stability and solubility.
Industrial Production Methods
In an industrial setting, the production of N4-Acetylcytidine triphosphate (sodium) involves large-scale chemical synthesis using high-performance liquid chromatography (HPLC) for purification. The process is optimized for yield and purity, ensuring that the compound meets the required standards for research and application.
Chemical Reactions Analysis
Types of Reactions
N4-Acetylcytidine triphosphate (sodium) undergoes various chemical reactions, including:
Oxidation: The compound can be oxidized under specific conditions, leading to the formation of oxidized derivatives.
Reduction: Reduction reactions can convert N4-Acetylcytidine triphosphate to its reduced forms, which may have different biological activities.
Substitution: The acetyl group at the N4 position can be substituted with other functional groups, altering the compound’s properties.
Common Reagents and Conditions
Oxidation: Common oxidizing agents include hydrogen peroxide and potassium permanganate.
Reduction: Sodium borohydride and lithium aluminum hydride are typical reducing agents.
Substitution: Various nucleophiles can be used for substitution reactions, depending on the desired product.
Major Products Formed
The major products formed from these reactions include oxidized and reduced derivatives of N4-Acetylcytidine triphosphate, as well as substituted analogs with different functional groups at the N4 position.
Scientific Research Applications
Role in RNA Modification and Stability
N4-Acetylcytidine (ac4C) is a critical modification found in RNA, particularly in mRNA, where it plays a vital role in enhancing translation efficiency and RNA stability. The presence of ac4C modifies the interaction between mRNA and ribosomes, thereby promoting protein synthesis.
- Mechanism : The acetylation of cytidine is catalyzed by the enzyme N-acetyltransferase 10 (NAT10) , which transfers an acetyl group from acetyl-CoA to the exocyclic N4-amine of cytidine. This modification is essential for the proper functioning of various cellular processes, including viral replication and tumor progression .
- Case Study : Research has shown that inhibiting NAT10 reduces the levels of ac4C in mRNA, leading to decreased viral replication in enterovirus 71 (EV71) and other RNA viruses such as HIV-1 and Zika virus. This indicates that targeting ac4C could be a potential therapeutic strategy against viral infections .
Applications in Cancer Research
Ac4CTP has been implicated in various cancer types, particularly through its association with NAT10. Elevated NAT10 levels have been linked to poor prognosis in cancers such as hepatocellular carcinoma (HCC) and acute myeloid leukemia (AML).
- Hepatocellular Carcinoma : Studies demonstrated that silencing NAT10 significantly inhibits HCC cell proliferation and metastasis by reducing ac4C levels, thereby affecting the translation of oncogenes like HMGB2 .
- Acute Myeloid Leukemia : In AML, NAT10-mediated ac4C enhances serine metabolism, promoting stem cell properties and leukemogenesis. This suggests that targeting ac4C could provide new avenues for treatment .
In Vitro Transcription Applications
Ac4CTP serves as an effective substrate for T7 RNA polymerase-catalyzed transcription in vitro. Its incorporation into RNA templates allows for the production of modified RNA molecules that can be utilized in various applications.
- Transcription Efficiency : The use of ac4CTP can enhance the yield of synthesized RNA, making it a valuable tool for producing high-quality RNA probes for research and diagnostic purposes .
Potential Therapeutic Applications
The unique properties of ac4CTP make it a candidate for developing novel therapeutics, particularly in the fields of virology and oncology.
- Viral Infections : By targeting the pathways involving ac4C modifications, researchers are exploring strategies to inhibit viral replication, providing a potential therapeutic angle against persistent viral infections .
- Cancer Treatment : The modulation of NAT10 activity and subsequent effects on ac4C levels could lead to innovative treatments for cancers characterized by high NAT10 expression .
Data Table: Summary of Applications
Mechanism of Action
N4-Acetylcytidine triphosphate (sodium) exerts its effects through the acetylation of RNA molecules. The acetyl group at the N4 position of cytidine enhances the stability and translation efficiency of RNA. This modification is catalyzed by the enzyme N-acetyltransferase 10 (NAT10), which transfers the acetyl group from acetyl-CoA to the N4 position of cytidine. The modified RNA molecules exhibit increased resistance to degradation and improved translation efficiency, contributing to the regulation of gene expression.
Comparison with Similar Compounds
Structural and Functional Overview
The following table summarizes key properties and applications of ac4CTP sodium compared to other nucleotide triphosphates:
*Estimated based on molecular formula.
Detailed Analysis
N4-Acetylcytidine Triphosphate (Sodium) vs. 5-Methylcytidine Triphosphate (5-Me-CTP)
- Structural Differences : ac4CTP sodium features an N4-acetyl group on cytidine, while 5-Me-CTP has a methyl group at the 5-position .
- Functional Contrast :
- Efficiency: Both compounds are efficiently incorporated by T7 RNA polymerase, but 5-Me-CTP is more widely adopted in therapeutic mRNA production due to its established role in reducing immunogenicity .
N4-Acetylcytidine Triphosphate (Sodium) vs. Deoxythymidine Triphosphate (dTTP)
- Role in Nucleic Acids :
- ac4CTP sodium is exclusive to RNA synthesis , while dTTP is a natural substrate for DNA polymerases .
Biological Activity
N4-Acetylcytidine triphosphate (sodium) is a modified nucleotide that has garnered attention for its biological activities, particularly in the context of mRNA modifications and their implications in various cellular processes. This article delves into the biological activity of N4-acetylcytidine triphosphate (ac4CTP), highlighting its role in gene expression regulation, effects on cellular metabolism, and potential therapeutic applications.
Overview of N4-Acetylcytidine Triphosphate
N4-acetylcytidine (ac4C) is an RNA modification catalyzed by the enzyme N-acetyltransferase 10 (NAT10). This modification has been implicated in the regulation of mRNA stability, translation, and interactions with various proteins. The presence of ac4C in mRNA can influence gene expression by altering the structural conformation of RNA, thereby enhancing or inhibiting translation depending on the context.
-
Translation Regulation :
- Ac4C enhances translation elongation by stabilizing interactions between mRNA and tRNAs, particularly in coding sequences (CDS). This stabilization occurs through intramolecular hydrogen bonding, which improves base-pairing with guanosine and inhibits co-translational decay pathways .
- In contrast, ac4C can negatively impact translation initiation under certain conditions by modifying base-pairing interactions in the 5' untranslated region (UTR) of mRNAs .
-
Impact on Cellular Metabolism :
- Recent studies have shown that ac4C plays a significant role in metabolic reprogramming, particularly in cancer cells. For instance, NAT10-mediated ac4C modification enhances serine uptake and biosynthesis in acute myeloid leukemia (AML), promoting stemness and progression of leukemia .
- In hepatocellular carcinoma (HCC), elevated levels of NAT10 and ac4C have been associated with poor patient outcomes. Silencing NAT10 significantly inhibits tumor growth by reducing ac4C levels, thereby affecting the translation of oncogenic proteins .
- Immunogenicity Modulation :
Table 1: Summary of Biological Activities of N4-Acetylcytidine Triphosphate
Case Studies
- Acute Myeloid Leukemia :
- Hepatocellular Carcinoma :
Q & A
Q. Basic: What experimental conditions optimize N4-acetylcytidine triphosphate (ac4CTP) sodium incorporation in T7 Polymerase-catalyzed transcription?
Methodological Answer:
- Substrate Concentration : Use 0.1–2 mM ac4CTP sodium in transcription reactions, as higher concentrations (e.g., 1–2 mM) enhance incorporation efficiency without inhibiting T7 Polymerase activity .
- Template Design : Ensure RNA templates contain cytidine residues at positions where acetylation is desired. Test templates with varying lengths and secondary structures to assess polymerase processivity .
- Buffer Conditions : Maintain pH 7.0–7.5 using phosphate-based buffers (e.g., 0.02 M potassium phosphate with 0.15 M KCl) to stabilize enzymatic activity .
Q. Advanced: How can researchers reconcile contradictory data on ac4CTP sodium’s dual role in cell growth inhibition and inflammatory signaling?
Methodological Answer:
- Dose-Dependent Studies : Perform time-course experiments (3–48 hours) with ac4CTP sodium concentrations ranging from 0.1–2 mM. Lower doses (0.1–0.3 mM) may favor transcription applications, while higher doses (>0.3 mM) trigger NFκB activation and HMGB1 upregulation in BV2 microglia .
- Pathway-Specific Inhibitors : Co-treat cells with NFκB inhibitors (e.g., BAY11-7082) to decouple transcriptional incorporation effects from inflammatory signaling .
- Multi-Omics Validation : Combine RNA-Seq (to assess ac4CTP incorporation fidelity) with proteomics (to quantify HMGB1/NLRP3 levels) .
Q. Basic: What analytical methods validate ac4CTP sodium incorporation into RNA transcripts?
Methodological Answer:
- Gel Electrophoresis : Resolve transcripts on denaturing urea-PAGE gels; acetylated cytidine may alter migration patterns compared to unmodified CTP .
- HPLC/MS : Use ion-pair reverse-phase HPLC coupled with mass spectrometry to detect acetylated nucleosides after RNA digestion with nuclease P1 .
- Antibody-Based Detection : Employ monoclonal antibodies (e.g., Clone 3C2C2) for dot-blot or ELISA to confirm ac4CTP incorporation in HeLa cell RNA isolates .
Q. Advanced: What downstream effects arise from ac4CTP sodium-induced HMGB1 upregulation in immune cells?
Methodological Answer:
- Co-Culture Models : Treat BV2 microglia with ac4CTP sodium (0.3–1 mM, 3–6 hours) and co-culture with neurons to assess HMGB1-mediated neuroinflammatory crosstalk .
- Chromatin Immunoprecipitation (ChIP) : Map NFκB binding sites in the HMGB1 promoter to confirm direct transcriptional regulation .
- In Vivo Validation : Inject ac4CTP sodium into animal models (e.g., zebrafish embryos) and quantify HMGB1 secretion via Western blot .
Q. Basic: How do buffer components affect ac4CTP sodium stability during in vitro transcription?
Methodological Answer:
- Cation Selection : Sodium ions (from ac4CTP sodium) may interfere with magnesium-dependent polymerase activity. Titrate MgCl₂ (2–6 mM) to balance enzyme efficiency and nucleotide stability .
- Reducing Agents : Avoid β-mercaptoethanol in buffers, as thiols can degrade acetylated nucleotides. Use DTT (1 mM) instead .
- Storage Conditions : Aliquot ac4CTP sodium in H₂O (≥125 mg/mL) and store at –80°C to prevent hydrolysis .
Q. Advanced: How does ac4CTP sodium compare to other modified CTP analogs (e.g., pseudouridine triphosphate) in mRNA stability and immunogenicity?
Methodological Answer:
- mRNA Half-Life Assays : Co-transcribe luciferase mRNA with ac4CTP sodium or pseudouridine triphosphate (Ψ-CTP) and measure decay rates in HEK293T cells using luciferase activity assays .
- Immune Profiling : Stimulate dendritic cells with modified mRNAs and quantify IFN-α/β secretion via ELISA. ac4CTP sodium may reduce TLR3/7/8 activation compared to unmodified CTP but less effectively than Ψ-CTP .
- Cryo-EM Structural Analysis : Resolve T7 Polymerase-ac4CTP complexes to compare steric effects with Ψ-CTP and predict incorporation fidelity .
Q. Basic: What controls are essential when designing experiments with ac4CTP sodium?
Methodological Answer:
- Negative Controls : Use unmodified CTP in parallel reactions to distinguish acetylation-specific effects.
- Enzyme Blanks : Omit T7 Polymerase to confirm transcription is enzyme-dependent.
- Purity Verification : Validate ac4CTP sodium purity via HPLC (≥95%) and test for endotoxin contamination if using in cell-based assays .
Q. Advanced: Can ac4CTP sodium be used to study epitranscriptomic regulation in prokaryotic systems?
Methodological Answer:
- Bacterial Polymerase Compatibility : Test E. coli RNA polymerase activity with ac4CTP sodium using in vitro transcription assays. Note that T7 Polymerase is more permissive to modifications than bacterial enzymes .
- Ribosome Profiling : Incorporate ac4CTP sodium into bacterial mRNA and perform ribo-seq to assess translation efficiency impacts .
- CRISPR Interference : Knock down putative acetyltransferases (e.g., RimI homologs) to probe endogenous ac4C modification pathways in prokaryotes .
Properties
IUPAC Name |
tetrasodium;[[[(2R,3S,4R,5R)-5-(4-acetamido-2-oxopyrimidin-1-yl)-3,4-dihydroxyoxolan-2-yl]methoxy-oxidophosphoryl]oxy-oxidophosphoryl] phosphate | |
---|---|---|
Details | Computed by Lexichem TK 2.7.0 (PubChem release 2021.05.07) | |
Source | PubChem | |
URL | https://pubchem.ncbi.nlm.nih.gov | |
Description | Data deposited in or computed by PubChem | |
InChI |
InChI=1S/C11H18N3O15P3.4Na/c1-5(15)12-7-2-3-14(11(18)13-7)10-9(17)8(16)6(27-10)4-26-31(22,23)29-32(24,25)28-30(19,20)21;;;;/h2-3,6,8-10,16-17H,4H2,1H3,(H,22,23)(H,24,25)(H2,19,20,21)(H,12,13,15,18);;;;/q;4*+1/p-4/t6-,8-,9-,10-;;;;/m1..../s1 | |
Details | Computed by InChI 1.0.6 (PubChem release 2021.05.07) | |
Source | PubChem | |
URL | https://pubchem.ncbi.nlm.nih.gov | |
Description | Data deposited in or computed by PubChem | |
InChI Key |
VSBLTNBKSQTQJC-XGZYCNACSA-J | |
Details | Computed by InChI 1.0.6 (PubChem release 2021.05.07) | |
Source | PubChem | |
URL | https://pubchem.ncbi.nlm.nih.gov | |
Description | Data deposited in or computed by PubChem | |
Canonical SMILES |
CC(=O)NC1=NC(=O)N(C=C1)C2C(C(C(O2)COP(=O)([O-])OP(=O)([O-])OP(=O)([O-])[O-])O)O.[Na+].[Na+].[Na+].[Na+] | |
Details | Computed by OEChem 2.3.0 (PubChem release 2021.05.07) | |
Source | PubChem | |
URL | https://pubchem.ncbi.nlm.nih.gov | |
Description | Data deposited in or computed by PubChem | |
Isomeric SMILES |
CC(=O)NC1=NC(=O)N(C=C1)[C@H]2[C@@H]([C@@H]([C@H](O2)COP(=O)([O-])OP(=O)([O-])OP(=O)([O-])[O-])O)O.[Na+].[Na+].[Na+].[Na+] | |
Details | Computed by OEChem 2.3.0 (PubChem release 2021.05.07) | |
Source | PubChem | |
URL | https://pubchem.ncbi.nlm.nih.gov | |
Description | Data deposited in or computed by PubChem | |
Molecular Formula |
C11H14N3Na4O15P3 | |
Details | Computed by PubChem 2.1 (PubChem release 2021.05.07) | |
Source | PubChem | |
URL | https://pubchem.ncbi.nlm.nih.gov | |
Description | Data deposited in or computed by PubChem | |
Molecular Weight |
613.12 g/mol | |
Details | Computed by PubChem 2.1 (PubChem release 2021.05.07) | |
Source | PubChem | |
URL | https://pubchem.ncbi.nlm.nih.gov | |
Description | Data deposited in or computed by PubChem | |
Disclaimer and Information on In-Vitro Research Products
Please be aware that all articles and product information presented on BenchChem are intended solely for informational purposes. The products available for purchase on BenchChem are specifically designed for in-vitro studies, which are conducted outside of living organisms. In-vitro studies, derived from the Latin term "in glass," involve experiments performed in controlled laboratory settings using cells or tissues. It is important to note that these products are not categorized as medicines or drugs, and they have not received approval from the FDA for the prevention, treatment, or cure of any medical condition, ailment, or disease. We must emphasize that any form of bodily introduction of these products into humans or animals is strictly prohibited by law. It is essential to adhere to these guidelines to ensure compliance with legal and ethical standards in research and experimentation.