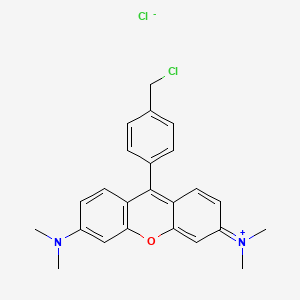
mitoTracker Orange
Overview
Description
MitoTracker Orange is a fluorescent dye used to stain mitochondria in live cells. It is a derivative of tetramethylrosamine and is known for its ability to accumulate in active mitochondria, making it a valuable tool for studying mitochondrial function and dynamics. The dye is well-retained after aldehyde fixation, which allows for further processing of the samples .
Preparation Methods
MitoTracker Orange is synthesized through a series of chemical reactions involving tetramethylrosamine derivatives. The synthetic route typically involves the introduction of a chloromethyl moiety to the rosamine structure, which imparts the dye with its mitochondrion-selective properties. The reaction conditions often include the use of organic solvents and mild temperatures to ensure the stability of the dye. Industrial production methods involve large-scale synthesis under controlled conditions to maintain the purity and efficacy of the dye .
Chemical Reactions Analysis
MitoTracker Orange undergoes several types of chemical reactions, including:
Oxidation: The dye can be oxidized to form different fluorescent species.
Reduction: It can be reduced to non-fluorescent forms, which can be re-oxidized to restore fluorescence.
Substitution: The chloromethyl group can react with thiols on proteins and peptides, forming stable conjugates.
Common reagents used in these reactions include oxidizing agents like hydrogen peroxide and reducing agents like sodium borohydride. The major products formed from these reactions are various fluorescent and non-fluorescent derivatives of the dye .
Scientific Research Applications
MitoTracker Orange is widely used in scientific research, particularly in the fields of:
Chemistry: It is used to study mitochondrial dynamics and function in various chemical environments.
Biology: The dye is employed to label and visualize mitochondria in live cells, aiding in the study of cellular metabolism, apoptosis, and other mitochondrial-related processes.
Medicine: this compound is used in medical research to investigate mitochondrial dysfunction in diseases such as cancer, neurodegenerative disorders, and metabolic syndromes.
Mechanism of Action
MitoTracker Orange exerts its effects by passively diffusing across the plasma membrane and accumulating in active mitochondria. The dye’s accumulation is dependent on the mitochondrial membrane potential, which allows it to selectively stain active mitochondria. Once inside the mitochondria, the chloromethyl group reacts with thiols on proteins and peptides, forming stable conjugates that are retained even after cell fixation .
Comparison with Similar Compounds
MitoTracker Orange is unique in its ability to be well-retained after aldehyde fixation, which is not a common feature among other mitochondrial dyes. Similar compounds include:
MitoTracker Red CMXRos: A derivative of X-rosamine, also used for mitochondrial staining but with different spectral properties.
MitoTracker Green FM: A carbocyanine-based dye that accumulates in active mitochondria but is not well-retained after fixation.
MitoTracker Deep Red FM: Another carbocyanine-based dye that is well-retained after fixation and used for multicolor labeling experiments
This compound stands out due to its specific spectral properties and its ability to form stable conjugates with mitochondrial proteins, making it a versatile tool for various research applications.
Properties
IUPAC Name |
[9-[4-(chloromethyl)phenyl]-6-(dimethylamino)xanthen-3-ylidene]-dimethylazanium;chloride | |
---|---|---|
Details | Computed by Lexichem TK 2.7.0 (PubChem release 2021.05.07) | |
Source | PubChem | |
URL | https://pubchem.ncbi.nlm.nih.gov | |
Description | Data deposited in or computed by PubChem | |
InChI |
InChI=1S/C24H24ClN2O.ClH/c1-26(2)18-9-11-20-22(13-18)28-23-14-19(27(3)4)10-12-21(23)24(20)17-7-5-16(15-25)6-8-17;/h5-14H,15H2,1-4H3;1H/q+1;/p-1 | |
Details | Computed by InChI 1.0.6 (PubChem release 2021.05.07) | |
Source | PubChem | |
URL | https://pubchem.ncbi.nlm.nih.gov | |
Description | Data deposited in or computed by PubChem | |
InChI Key |
FZTMEYOUQQFBJR-UHFFFAOYSA-M | |
Details | Computed by InChI 1.0.6 (PubChem release 2021.05.07) | |
Source | PubChem | |
URL | https://pubchem.ncbi.nlm.nih.gov | |
Description | Data deposited in or computed by PubChem | |
Canonical SMILES |
CN(C)C1=CC2=C(C=C1)C(=C3C=CC(=[N+](C)C)C=C3O2)C4=CC=C(C=C4)CCl.[Cl-] | |
Details | Computed by OEChem 2.3.0 (PubChem release 2021.05.07) | |
Source | PubChem | |
URL | https://pubchem.ncbi.nlm.nih.gov | |
Description | Data deposited in or computed by PubChem | |
Molecular Formula |
C24H24Cl2N2O | |
Details | Computed by PubChem 2.1 (PubChem release 2021.05.07) | |
Source | PubChem | |
URL | https://pubchem.ncbi.nlm.nih.gov | |
Description | Data deposited in or computed by PubChem | |
Molecular Weight |
427.4 g/mol | |
Details | Computed by PubChem 2.1 (PubChem release 2021.05.07) | |
Source | PubChem | |
URL | https://pubchem.ncbi.nlm.nih.gov | |
Description | Data deposited in or computed by PubChem | |
CAS No. |
199116-50-2 | |
Record name | Xanthylium, 9-[4-(chloromethyl)phenyl]-3,6-bis(dimethylamino)-, chloride (1:1) | |
Source | CAS Common Chemistry | |
URL | https://commonchemistry.cas.org/detail?cas_rn=199116-50-2 | |
Description | CAS Common Chemistry is an open community resource for accessing chemical information. Nearly 500,000 chemical substances from CAS REGISTRY cover areas of community interest, including common and frequently regulated chemicals, and those relevant to high school and undergraduate chemistry classes. This chemical information, curated by our expert scientists, is provided in alignment with our mission as a division of the American Chemical Society. | |
Explanation | The data from CAS Common Chemistry is provided under a CC-BY-NC 4.0 license, unless otherwise stated. | |
Retrosynthesis Analysis
AI-Powered Synthesis Planning: Our tool employs the Template_relevance Pistachio, Template_relevance Bkms_metabolic, Template_relevance Pistachio_ringbreaker, Template_relevance Reaxys, Template_relevance Reaxys_biocatalysis model, leveraging a vast database of chemical reactions to predict feasible synthetic routes.
One-Step Synthesis Focus: Specifically designed for one-step synthesis, it provides concise and direct routes for your target compounds, streamlining the synthesis process.
Accurate Predictions: Utilizing the extensive PISTACHIO, BKMS_METABOLIC, PISTACHIO_RINGBREAKER, REAXYS, REAXYS_BIOCATALYSIS database, our tool offers high-accuracy predictions, reflecting the latest in chemical research and data.
Strategy Settings
Precursor scoring | Relevance Heuristic |
---|---|
Min. plausibility | 0.01 |
Model | Template_relevance |
Template Set | Pistachio/Bkms_metabolic/Pistachio_ringbreaker/Reaxys/Reaxys_biocatalysis |
Top-N result to add to graph | 6 |
Feasible Synthetic Routes
Disclaimer and Information on In-Vitro Research Products
Please be aware that all articles and product information presented on BenchChem are intended solely for informational purposes. The products available for purchase on BenchChem are specifically designed for in-vitro studies, which are conducted outside of living organisms. In-vitro studies, derived from the Latin term "in glass," involve experiments performed in controlled laboratory settings using cells or tissues. It is important to note that these products are not categorized as medicines or drugs, and they have not received approval from the FDA for the prevention, treatment, or cure of any medical condition, ailment, or disease. We must emphasize that any form of bodily introduction of these products into humans or animals is strictly prohibited by law. It is essential to adhere to these guidelines to ensure compliance with legal and ethical standards in research and experimentation.