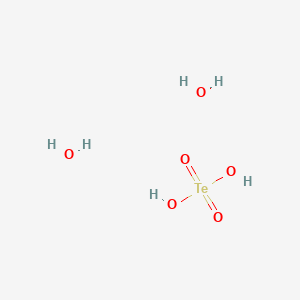
Telluric acid dihydrate
- Click on QUICK INQUIRY to receive a quote from our team of experts.
- With the quality product at a COMPETITIVE price, you can focus more on your research.
Overview
Description
Telluric acid dihydrate, with the chemical formula H6TeO6·2H2O, is a compound of tellurium, hydrogen, and oxygen. It is a white crystalline solid that is highly soluble in water. Telluric acid is the highest oxidized form of tellurium, a chemical element in the chalcogen group, which also includes oxygen, sulfur, selenium, and polonium. This compound is known for its strong oxidizing properties and its ability to form various tellurates.
Mechanism of Action
Target of Action
Telluric acid dihydrate primarily targets the synthesis of tellurium-based compounds . These compounds are known for their semiconducting behavior, making them useful in electronic devices .
Mode of Action
this compound is a weak acid that fully ionizes in solution . It reacts with strong bases to form tellurate salts and hydrogen tellurate salts with weaker bases or upon hydrolysis of tellurates in water . It is also used as a tellurium source in the synthesis of oxidation catalysts .
Biochemical Pathways
this compound has been shown to affect the Toll-like receptor-4 (TLR4) cascade, phosphoinositide 3-kinase (PI3K)/Akt axis, and nuclear erythroid-related factor-2 (Nrf-2) pathway . These pathways play crucial roles in inflammation and oxidative stress responses.
Pharmacokinetics
It is known that the compound exhibits the unusual property of sublimation when heated, transitioning directly from a solid to a gas without first becoming a liquid . This could potentially impact its bioavailability.
Result of Action
this compound has been used in research as a precursor for the synthesis of tellurium-based compounds . Some of these compounds exhibit semiconducting behavior, making them useful in electronic devices . In addition, telluric acid and its salts, tellurates, are known for their powerful oxidizing properties .
Action Environment
Environmental factors such as temperature can influence its stability, as it can sublimate when heated .
Preparation Methods
Synthetic Routes and Reaction Conditions: Telluric acid dihydrate is typically prepared by the oxidation of tellurium or tellurium dioxide with strong oxidizing agents such as hydrogen peroxide, chromium trioxide, or sodium peroxide. The reaction can be summarized as follows: [ \text{TeO}_2 + \text{H}_2\text{O}_2 + 2 \text{H}_2\text{O} \rightarrow \text{Te(OH)}_6 ] Crystallization of telluric acid solutions below 10°C results in the formation of telluric acid tetrahydrate, which can be further processed to obtain the dihydrate form .
Industrial Production Methods: In industrial settings, this compound is produced by similar oxidation processes, often involving large-scale reactors and controlled conditions to ensure high purity and yield. The use of hydrogen peroxide as an oxidizing agent is common due to its effectiveness and availability.
Chemical Reactions Analysis
Types of Reactions: Telluric acid dihydrate undergoes various chemical reactions, including:
Oxidation: It acts as a powerful oxidizing agent, capable of oxidizing other substances.
Reduction: It can be reduced to lower oxidation states of tellurium, such as tellurous acid.
Substitution: It can form tellurate salts with strong bases and hydrogen tellurate salts with weaker bases.
Common Reagents and Conditions:
Oxidation: Hydrogen peroxide, chromium trioxide, sodium peroxide.
Reduction: Hydrazine, potassium thiocyanate.
Substitution: Strong bases like sodium hydroxide, weaker bases like ammonium hydroxide.
Major Products:
Oxidation: Tellurium dioxide, various tellurates.
Reduction: Tellurous acid, elemental tellurium.
Substitution: Sodium tellurate, ammonium tellurate.
Scientific Research Applications
Telluric acid dihydrate has several scientific research applications:
Medicine: Its strong oxidizing properties are being explored for potential therapeutic applications.
Comparison with Similar Compounds
Sulfuric acid (H2SO4): Both are strong acids and oxidizing agents, but sulfuric acid is more commonly used and has a broader range of applications.
Selenic acid (H2SeO4): Similar in structure and properties, but selenic acid is less stable and less commonly used.
Teflic acid (H5IO6): Another strong oxidizing agent, but with different chemical properties and applications.
Uniqueness: Telluric acid dihydrate is unique due to its high oxidation state and strong oxidizing properties. It is less commonly encountered than sulfuric or selenic acids, making it a specialized compound for specific research and industrial applications.
Properties
IUPAC Name |
telluric acid;dihydrate |
Source
|
---|---|---|
Details | Computed by Lexichem TK 2.7.0 (PubChem release 2021.05.07) | |
Source | PubChem | |
URL | https://pubchem.ncbi.nlm.nih.gov | |
Description | Data deposited in or computed by PubChem | |
InChI |
InChI=1S/H2O4Te.2H2O/c1-5(2,3)4;;/h(H2,1,2,3,4);2*1H2 |
Source
|
Details | Computed by InChI 1.0.6 (PubChem release 2021.05.07) | |
Source | PubChem | |
URL | https://pubchem.ncbi.nlm.nih.gov | |
Description | Data deposited in or computed by PubChem | |
InChI Key |
VCYUGZHEVVXOPD-UHFFFAOYSA-N |
Source
|
Details | Computed by InChI 1.0.6 (PubChem release 2021.05.07) | |
Source | PubChem | |
URL | https://pubchem.ncbi.nlm.nih.gov | |
Description | Data deposited in or computed by PubChem | |
Canonical SMILES |
O.O.O[Te](=O)(=O)O |
Source
|
Details | Computed by OEChem 2.3.0 (PubChem release 2021.05.07) | |
Source | PubChem | |
URL | https://pubchem.ncbi.nlm.nih.gov | |
Description | Data deposited in or computed by PubChem | |
Molecular Formula |
H6O6Te |
Source
|
Details | Computed by PubChem 2.1 (PubChem release 2021.05.07) | |
Source | PubChem | |
URL | https://pubchem.ncbi.nlm.nih.gov | |
Description | Data deposited in or computed by PubChem | |
Molecular Weight |
229.6 g/mol |
Source
|
Details | Computed by PubChem 2.1 (PubChem release 2021.05.07) | |
Source | PubChem | |
URL | https://pubchem.ncbi.nlm.nih.gov | |
Description | Data deposited in or computed by PubChem | |
Retrosynthesis Analysis
AI-Powered Synthesis Planning: Our tool employs the Template_relevance Pistachio, Template_relevance Bkms_metabolic, Template_relevance Pistachio_ringbreaker, Template_relevance Reaxys, Template_relevance Reaxys_biocatalysis model, leveraging a vast database of chemical reactions to predict feasible synthetic routes.
One-Step Synthesis Focus: Specifically designed for one-step synthesis, it provides concise and direct routes for your target compounds, streamlining the synthesis process.
Accurate Predictions: Utilizing the extensive PISTACHIO, BKMS_METABOLIC, PISTACHIO_RINGBREAKER, REAXYS, REAXYS_BIOCATALYSIS database, our tool offers high-accuracy predictions, reflecting the latest in chemical research and data.
Strategy Settings
Precursor scoring | Relevance Heuristic |
---|---|
Min. plausibility | 0.01 |
Model | Template_relevance |
Template Set | Pistachio/Bkms_metabolic/Pistachio_ringbreaker/Reaxys/Reaxys_biocatalysis |
Top-N result to add to graph | 6 |
Feasible Synthetic Routes
Disclaimer and Information on In-Vitro Research Products
Please be aware that all articles and product information presented on BenchChem are intended solely for informational purposes. The products available for purchase on BenchChem are specifically designed for in-vitro studies, which are conducted outside of living organisms. In-vitro studies, derived from the Latin term "in glass," involve experiments performed in controlled laboratory settings using cells or tissues. It is important to note that these products are not categorized as medicines or drugs, and they have not received approval from the FDA for the prevention, treatment, or cure of any medical condition, ailment, or disease. We must emphasize that any form of bodily introduction of these products into humans or animals is strictly prohibited by law. It is essential to adhere to these guidelines to ensure compliance with legal and ethical standards in research and experimentation.