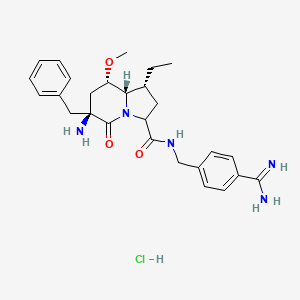
Stainless steel powder, type 316
Overview
Description
Stainless steel powder, type 316L (SS316L), is an austenitic stainless steel variant optimized for additive manufacturing (AM) and powder metallurgy. Its composition includes 16–18% chromium (Cr), 10–14% nickel (Ni), 2–3% molybdenum (Mo), and a low carbon content (≤0.03% C) to minimize carbide precipitation during welding . Key properties include:
- Corrosion resistance: Superior to standard 316 due to Mo, which enhances resistance to pitting and crevice corrosion in chloride-rich environments .
- Weldability: Reduced risk of sensitization (intergranular corrosion) due to low carbon content .
- High-temperature stability: Retains strength and oxidation resistance up to 800°C .
- Biocompatibility: Widely used in medical implants .
Applications span aerospace, marine, chemical processing, and biomedical industries, where complex geometries and corrosion resistance are critical .
Preparation Methods
Synthetic Routes and Reaction Conditions: Stainless steel powder, type 316 is typically produced using Inert Gas Atomization (IGA). This process involves melting the alloy and then atomizing it into fine droplets using an inert gas, such as argon. The droplets solidify into spherical particles, which are then collected as powder. The IGA process ensures a low oxygen content and low impurity levels, resulting in a clean product with enhanced mechanical performance .
Industrial Production Methods: In industrial settings, this compound is produced using various methods, including:
Hot Isostatic Pressing (HIP): This method involves applying high pressure and temperature to the powder to form dense, solid parts.
Metal Injection Moulding (MIM): This process mixes the powder with a binder to create a feedstock, which is then injected into molds to form complex shapes.
Laser Powder Bed Fusion (LPBF): This additive manufacturing technique uses a laser to selectively melt and fuse the powder layer by layer to create 3D parts.
Chemical Reactions Analysis
Types of Reactions: Stainless steel powder, type 316 undergoes various chemical reactions, including:
Oxidation: The alloy forms a passive oxide layer on its surface, which protects it from further corrosion.
Reduction: In reducing environments, the oxide layer can be reduced, exposing the underlying metal.
Substitution: Alloying elements such as chromium and nickel can substitute for iron in the crystal lattice, enhancing corrosion resistance.
Common Reagents and Conditions:
Oxidizing Agents: Nitric acid and oxygen are commonly used to oxidize the alloy.
Reducing Agents: Hydrogen and carbon monoxide can reduce the oxide layer.
Substitution Reactions: Alloying elements are added during the melting process to achieve the desired composition.
Major Products Formed:
Oxide Layers: Chromium oxide and nickel oxide are the primary products of oxidation.
Reduced Metal: Pure iron, chromium, and nickel are formed during reduction reactions.
Scientific Research Applications
-
Additive Manufacturing
- 3D Printing : Type 316 stainless steel powder is extensively used in laser-based additive manufacturing processes, such as Selective Laser Melting (SLM) and Electron Beam Melting (EBM). Its high corrosion resistance and mechanical properties make it ideal for producing complex geometries and lightweight components in aerospace and automotive industries .
- Biomedical Devices : Due to its biocompatibility, 316 stainless steel powder is favored for manufacturing medical implants and surgical instruments. Its resistance to bodily fluids ensures durability and safety .
- Automotive Components
- Chemical Processing Equipment
- Marine Applications
- Food Processing
Case Studies Highlighting Applications
-
Case Study 1: Pressure Sensors
Intech Powdered Metal utilized fully dense 316 stainless steel to combine multiple machined parts into one component for a pressure sensor manufacturer. This approach not only met corrosion resistance requirements but also reduced material costs by half compared to traditional machining methods . -
Case Study 2: Sporting Goods
A sporting goods company required a strong yet irregularly shaped component. Intech's solution involved using fully dense 316 stainless steel, which successfully met the strength and corrosion testing requirements while optimizing the design . -
Case Study 3: Tamper-Proof Hardware
A client needed a tamper-proof nut that could withstand significant force without breaking. By tailoring the manufacturing process with 316 stainless steel powder, Intech provided a solution that met all mechanical requirements while ensuring high corrosion resistance .
Advantages of Using Stainless Steel Powder Type 316
- Design Flexibility : The powder form allows for the creation of complex shapes that are difficult to achieve with traditional machining.
- Cost Efficiency : Reduced material waste and the ability to combine multiple components into one part can lead to significant cost savings.
- Enhanced Properties : The unique properties of type 316 stainless steel provide superior wear resistance and longevity in demanding applications.
Mechanism of Action
The primary mechanism by which Stainless steel powder, type 316 exerts its effects is through the formation of a passive oxide layer on its surface. This layer, primarily composed of chromium oxide, acts as a barrier to prevent further oxidation and corrosion. The alloying elements, such as chromium and nickel, enhance the stability and durability of this oxide layer. Additionally, the low carbon content in 304L Stainless Steel minimizes carbide precipitation, which can otherwise lead to intergranular corrosion .
Comparison with Similar Compounds
Chemical Composition
SS316L is distinguished by its Mo content and low carbon compared to other austenitic and duplex stainless steels:
Grade | Cr (%) | Ni (%) | Mo (%) | C (%) | Key Additives |
---|---|---|---|---|---|
316L | 16–18 | 10–14 | 2–3 | ≤0.03 | – |
316 | 16–18 | 10–14 | 2–3 | ≤0.08 | – |
316H | 16–18 | 10–14 | 2–3 | 0.04–0.10 | Higher carbon |
316Ti | 16–18 | 10–14 | 2–3 | ≤0.08 | Titanium (Ti) |
304/304L | 18–20 | 8–12 | – | ≤0.03–0.08 | – |
Duplex 2205 | 22–23 | 4.5–6.5 | 3–3.5 | ≤0.03 | Nitrogen (N) |
Key Observations :
- 316 vs. 316L : 316L’s lower carbon (≤0.03% vs. 0.08%) improves weldability and reduces sensitization .
- 316Ti : Titanium stabilizes carbides, enhancing high-temperature strength and chloride resistance .
- 304/304L : Lacks Mo, making it less suitable for marine or chemical environments .
Mechanical and Corrosion Properties
Strength and Wear Resistance:
- SS316L : Tensile strength ranges from 485–620 MPa (as-printed) . Adding 5–10% Mo particles increases yield strength by 15–20% and wear resistance by 30% .
- Duplex 2205 : Higher strength (620–750 MPa) due to ferritic-austenitic microstructure but lower ductility .
- 316H : Higher creep resistance at elevated temperatures due to carbon content .
Corrosion Resistance:
- Pitting Resistance Equivalent (PRE) : SS316L has PRE = Cr + 3.3Mo + 16N ≈ 26–30, outperforming 304 (PRE ≈ 18–20) .
- Mo Additives : 5 wt.% Mo in SS316L reduces corrosion current density by two orders of magnitude in acidic environments .
- Duplex Steels : Superior resistance to stress corrosion cracking but vulnerable to sigma phase formation above 600°C .
Manufacturing Behavior
Powder Characteristics:
- Particle Size : SS316L powders for AM typically range 15–45 µm, with spherical morphology for optimal flowability .
- Density : SS316L parts achieve >99% density with laser powder bed fusion (LPBF) at 30–45 µm layer thickness .
Powder Grade | Layer Thickness (µm) | Relative Density (%) | Key Defects |
---|---|---|---|
316L | 30 | 99.5 | Minor porosity |
316L + TiC | 30 | 98.8 | Unmelted TiC particles |
304L | 45 | 98.2 | Higher porosity |
Post-Processing : SS316L requires stress-relief annealing to mitigate residual thermal stresses from AM .
Cost and Availability
Biological Activity
Stainless steel powder, particularly type 316, is widely recognized for its excellent mechanical properties and corrosion resistance. However, its biological activity, especially in medical and biomedical applications, has become a significant area of research. This article delves into the biological activity of 316 stainless steel powder, focusing on its antibacterial properties, cytotoxicity, and applications in implantable devices.
Composition and Properties of Stainless Steel 316
Stainless steel type 316 is an austenitic alloy primarily composed of iron, chromium, nickel, and molybdenum. Its typical composition includes:
Element | Weight % | Role |
---|---|---|
Iron (Fe) | Balance | Base metal |
Chromium (Cr) | 16-18% | Corrosion resistance |
Nickel (Ni) | 10-14% | Corrosion resistance |
Molybdenum (Mo) | 2-3% | Pitting resistance |
The presence of chromium provides inherent corrosion resistance, while molybdenum enhances resistance to pitting corrosion, particularly in chloride-rich environments such as seawater .
Antibacterial Activity
Recent studies have highlighted the antibacterial properties of modified stainless steel 316L. For instance, research on copper-bearing 316L stainless steel demonstrated a broad-spectrum antibacterial effect against pathogens such as Staphylococcus aureus, Escherichia coli, and Staphylococcus epidermidis. The copper-doped variant exhibited bacterial reduction percentages of approximately 95% for these strains .
Case Study: Copper-Bearing 316L Stainless Steel
- Objective : To evaluate the antibacterial efficacy of copper-doped 316L stainless steel.
- Method : In vitro assays were conducted to assess bacterial reduction.
- Results : The study found that the modified stainless steel significantly reduced biofilm formation and bacterial counts compared to standard 316L stainless steel. In vivo tests on rat models further confirmed these findings, showing reduced infection rates associated with implants made from copper-bearing alloys .
Cytotoxicity Assessment
The cytotoxicity of stainless steel powders is crucial for their application in medical implants. Research indicates that while pure 316L stainless steel exhibits low cytotoxicity, modifications (such as alloying with boron or titanium) can influence its biological response.
Study Findings
A study assessed various formulations of modified 316L stainless steel regarding their cytotoxic effects. The results indicated that:
- Pure 316L had minimal cytotoxic effects.
- Boron and titanium additions altered cell viability positively but required careful optimization to balance mechanical properties and biocompatibility .
Applications in Biomedical Devices
The unique properties of stainless steel type 316 make it suitable for various biomedical applications, including:
- Implants : Used in orthopedic devices due to its strength and corrosion resistance.
- Surgical instruments : Preferred for their durability and ease of sterilization.
- Dental applications : Utilized in dental implants where biocompatibility is critical.
Q & A
Basic Research Questions
Q. What methodologies are recommended for characterizing the particle size distribution and morphology of 316L stainless steel powder in additive manufacturing (AM)?
Methodological Answer: Use scanning electron microscopy (SEM) to analyze particle morphology, noting deviations from sphericity and surface defects (e.g., satellites or cracks) . Laser diffraction or dynamic image analysis quantifies particle size distribution (PSD), with D10, D50, and D90 values critical for flowability and packing density . For recycled powders, compare PSD shifts (e.g., increased fines due to agglomeration) and surface roughness via atomic force microscopy (AFM) .
Q. How are corrosion resistance properties of 316L stainless steel powder validated in acidic environments?
Methodological Answer: Conduct electrochemical tests (e.g., potentiodynamic polarization, electrochemical impedance spectroscopy) per ASTM G5/G59 standards to assess pitting resistance in chloride-rich or acidic media (e.g., H2SO4, HCl). Immersion tests in simulated industrial environments (e.g., marine conditions) quantify mass loss and surface degradation over time . X-ray photoelectron spectroscopy (XPS) identifies oxide layer composition (e.g., Cr2O3/Fe3O4 ratios) influencing passivation .
Q. What standards govern the chemical composition and impurity analysis of 316L powder for research reproducibility?
Methodological Answer: Refer to ASTM A276/A479 for compositional limits (e.g., Cr: 16–18%, Mo: 2–3%, C ≤0.03%) . Inductively coupled plasma mass spectrometry (ICP-MS) quantifies trace elements (e.g., O, N, S), while inert gas fusion (IGA) measures interstitial impurities. Cross-check with SEM-EDS for localized segregation .
Advanced Research Questions
Q. How do laser parameters in selective laser melting (SLM) influence the dynamic mechanical properties of 316L stainless steel?
Methodological Answer: Optimize laser power (150–400 W), scan speed (500–1500 mm/s), and hatch spacing (50–150 µm) to balance energy density (e.g., 50–100 J/mm³) and minimize defects like porosity . Use split-Hopkinson pressure bar (SHPB) tests to evaluate high-strain-rate behavior (10<sup>3</sup>–10<sup>4</sup> s<sup>−1</sup>). Modify the Johnson-Cook constitutive model to account for strain-rate hardening and thermal softening . Validate with microstructural analysis (EBSD/TEM) of dislocation networks .
Q. What experimental approaches reconcile contradictions in recycled 316L powder behavior (e.g., surface roughness vs. oxidation)?
Methodological Answer: Combine multi-scale techniques:
- AFM : Quantify reduced surface roughness (12 nm vs. 25 nm in virgin powder) due to dendrite loss .
- HAXPES : Detect increased Fe<sup>2+</sup>/Cr<sup>1+</sup> oxidation states and MnSiO3 formation at deeper sampling depths (~20 nm) .
- Nanoindentation : Measure hardness increases (e.g., 3.2 GPa vs. 2.9 GPa) from oxide-induced work hardening . Cross-reference with flowability tests (Hall flowmeter) to assess practical usability .
Q. How does thermal annealing affect the microstructure and thermal stability of LPBF-produced 316L?
Methodological Answer: Anneal at 800–1050°C for 1–4 hours. Use electron backscatter diffraction (EBSD) to track cellular structure dissolution at ≥800°C and retained austenite stability . Differential scanning calorimetry (DSC) identifies phase transitions, while nanoindentation maps hardness reductions (e.g., from 250 HV to 180 HV) due to dislocation recovery . Compare with JMatPro-simulated phase equilibria .
Q. What numerical models predict densification behavior of 316L powder during hot isostatic pressing (HIP)?
Methodological Answer: Implement finite element modeling (FEM) with JMatPro-derived flow stress data at varying temperatures (20–1200°C) . Calibrate creep parameters (e.g., Norton exponent) using dilatometry. Validate via micro-CT to quantify pore closure efficiency (>99.5% density) .
Properties
IUPAC Name |
(1R,6S,8S,8aS)-6-amino-6-benzyl-N-[(4-carbamimidoylphenyl)methyl]-1-ethyl-8-methoxy-5-oxo-1,2,3,7,8,8a-hexahydroindolizine-3-carboxamide;hydrochloride | |
---|---|---|
Details | Computed by Lexichem TK 2.7.0 (PubChem release 2021.05.07) | |
Source | PubChem | |
URL | https://pubchem.ncbi.nlm.nih.gov | |
Description | Data deposited in or computed by PubChem | |
InChI |
InChI=1S/C27H35N5O3.ClH/c1-3-19-13-21(25(33)31-16-18-9-11-20(12-10-18)24(28)29)32-23(19)22(35-2)15-27(30,26(32)34)14-17-7-5-4-6-8-17;/h4-12,19,21-23H,3,13-16,30H2,1-2H3,(H3,28,29)(H,31,33);1H/t19-,21?,22+,23+,27+;/m1./s1 | |
Details | Computed by InChI 1.0.6 (PubChem release 2021.05.07) | |
Source | PubChem | |
URL | https://pubchem.ncbi.nlm.nih.gov | |
Description | Data deposited in or computed by PubChem | |
InChI Key |
HDSUFZKBUUJDGC-MMVKSQEVSA-N | |
Details | Computed by InChI 1.0.6 (PubChem release 2021.05.07) | |
Source | PubChem | |
URL | https://pubchem.ncbi.nlm.nih.gov | |
Description | Data deposited in or computed by PubChem | |
Canonical SMILES |
CCC1CC(N2C1C(CC(C2=O)(CC3=CC=CC=C3)N)OC)C(=O)NCC4=CC=C(C=C4)C(=N)N.Cl | |
Details | Computed by OEChem 2.3.0 (PubChem release 2021.05.07) | |
Source | PubChem | |
URL | https://pubchem.ncbi.nlm.nih.gov | |
Description | Data deposited in or computed by PubChem | |
Isomeric SMILES |
CC[C@@H]1CC(N2[C@@H]1[C@H](C[C@](C2=O)(CC3=CC=CC=C3)N)OC)C(=O)NCC4=CC=C(C=C4)C(=N)N.Cl | |
Details | Computed by OEChem 2.3.0 (PubChem release 2021.05.07) | |
Source | PubChem | |
URL | https://pubchem.ncbi.nlm.nih.gov | |
Description | Data deposited in or computed by PubChem | |
Molecular Formula |
C27H36ClN5O3 | |
Details | Computed by PubChem 2.1 (PubChem release 2021.05.07) | |
Source | PubChem | |
URL | https://pubchem.ncbi.nlm.nih.gov | |
Description | Data deposited in or computed by PubChem | |
Molecular Weight |
514.1 g/mol | |
Details | Computed by PubChem 2.1 (PubChem release 2021.05.07) | |
Source | PubChem | |
URL | https://pubchem.ncbi.nlm.nih.gov | |
Description | Data deposited in or computed by PubChem | |
Retrosynthesis Analysis
AI-Powered Synthesis Planning: Our tool employs the Template_relevance Pistachio, Template_relevance Bkms_metabolic, Template_relevance Pistachio_ringbreaker, Template_relevance Reaxys, Template_relevance Reaxys_biocatalysis model, leveraging a vast database of chemical reactions to predict feasible synthetic routes.
One-Step Synthesis Focus: Specifically designed for one-step synthesis, it provides concise and direct routes for your target compounds, streamlining the synthesis process.
Accurate Predictions: Utilizing the extensive PISTACHIO, BKMS_METABOLIC, PISTACHIO_RINGBREAKER, REAXYS, REAXYS_BIOCATALYSIS database, our tool offers high-accuracy predictions, reflecting the latest in chemical research and data.
Strategy Settings
Precursor scoring | Relevance Heuristic |
---|---|
Min. plausibility | 0.01 |
Model | Template_relevance |
Template Set | Pistachio/Bkms_metabolic/Pistachio_ringbreaker/Reaxys/Reaxys_biocatalysis |
Top-N result to add to graph | 6 |
Feasible Synthetic Routes
Disclaimer and Information on In-Vitro Research Products
Please be aware that all articles and product information presented on BenchChem are intended solely for informational purposes. The products available for purchase on BenchChem are specifically designed for in-vitro studies, which are conducted outside of living organisms. In-vitro studies, derived from the Latin term "in glass," involve experiments performed in controlled laboratory settings using cells or tissues. It is important to note that these products are not categorized as medicines or drugs, and they have not received approval from the FDA for the prevention, treatment, or cure of any medical condition, ailment, or disease. We must emphasize that any form of bodily introduction of these products into humans or animals is strictly prohibited by law. It is essential to adhere to these guidelines to ensure compliance with legal and ethical standards in research and experimentation.