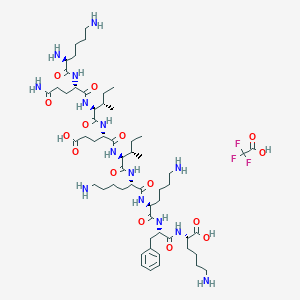
Gap19 (TFA)
- Click on QUICK INQUIRY to receive a quote from our team of experts.
- With the quality product at a COMPETITIVE price, you can focus more on your research.
Overview
Description
Gap19 (TFA) is a peptide derived from nine amino acids of the cytoplasmic loop of connexin 43 (Cx43). It is a potent and selective blocker of Cx43 hemichannels, which are involved in various physiological and pathological processes. Gap19 (TFA) is known for its ability to prevent the intramolecular interactions between the C-terminus and the cytoplasmic loop of Cx43, thereby inhibiting hemichannel activity without affecting gap junction channels .
Preparation Methods
Synthetic Routes and Reaction Conditions
Gap19 (TFA) is synthesized using solid-phase peptide synthesis (SPPS), a common method for producing peptides. The synthesis involves the sequential addition of protected amino acids to a growing peptide chain anchored to a solid resin. The process typically includes the following steps:
Resin Loading: The first amino acid is attached to the resin.
Deprotection: The protecting group of the amino acid is removed.
Coupling: The next amino acid, with its protecting group, is added and coupled to the growing chain.
Repetition: Steps 2 and 3 are repeated until the desired peptide sequence is complete.
Cleavage: The peptide is cleaved from the resin and deprotected to yield the final product.
Industrial Production Methods
Industrial production of Gap19 (TFA) follows similar principles but on a larger scale. Automated peptide synthesizers are often used to increase efficiency and consistency. The process is optimized to ensure high purity and yield, with rigorous quality control measures in place to verify the final product’s integrity .
Chemical Reactions Analysis
Types of Reactions
Gap19 (TFA) primarily undergoes peptide bond formation during its synthesis. It does not typically participate in oxidation, reduction, or substitution reactions under normal conditions. it can interact with other molecules through non-covalent interactions such as hydrogen bonding and hydrophobic interactions .
Common Reagents and Conditions
Reagents: Protected amino acids, coupling reagents (e.g., HBTU, DIC), deprotecting agents (e.g., TFA), and solvents (e.g., DMF, DCM).
Conditions: Room temperature for coupling reactions, acidic conditions for deprotection and cleavage.
Major Products
The major product of the synthesis is the Gap19 (TFA) peptide itself. By-products may include truncated peptides or peptides with incomplete deprotection, which are typically removed through purification processes such as HPLC .
Scientific Research Applications
Gap19 (TFA) has a wide range of applications in scientific research:
Cardiology: It is used to study the role of Cx43 hemichannels in cardiac ischemia/reperfusion injury and to develop potential therapeutic strategies for heart diseases
Neuroscience: Gap19 (TFA) is employed to investigate neuroglial interactions and the role of hemichannels in neuronal activity and survival.
Cell Biology: Researchers use Gap19 (TFA) to explore cell communication mechanisms and the regulation of hemichannel activity in various cell types.
Pharmacology: It serves as a tool to screen for new drugs targeting Cx43 hemichannels and to understand the pharmacodynamics of hemichannel blockers.
Mechanism of Action
Gap19 (TFA) exerts its effects by binding to the C-terminus of Cx43, preventing its interaction with the cytoplasmic loop. This inhibition blocks the opening of hemichannels, thereby reducing the exchange of ions and metabolites between the cytoplasm and the extracellular environment. This mechanism is particularly beneficial in conditions where uncontrolled hemichannel activity leads to cellular damage, such as during ischemia/reperfusion injury .
Comparison with Similar Compounds
Gap19 (TFA) is unique in its selective inhibition of Cx43 hemichannels without affecting gap junction channels. Similar compounds include:
Gap26 and Gap27: These peptides also target Cx43 but can inhibit both hemichannels and gap junction channels.
Carbenoxolone: A non-specific hemichannel blocker that affects multiple connexin types and gap junctions.
TAT-Gap19: A modified version of Gap19 with enhanced cell permeability, used in studies requiring intracellular delivery.
Gap19 (TFA) stands out due to its specificity and effectiveness in blocking hemichannels, making it a valuable tool in research and potential therapeutic applications .
Properties
IUPAC Name |
(2S)-6-amino-2-[[(2S)-2-[[(2S)-6-amino-2-[[(2S)-6-amino-2-[[(2S,3S)-2-[[(2S)-2-[[(2S,3S)-2-[[(2S)-5-amino-2-[[(2S)-2,6-diaminohexanoyl]amino]-5-oxopentanoyl]amino]-3-methylpentanoyl]amino]-4-carboxybutanoyl]amino]-3-methylpentanoyl]amino]hexanoyl]amino]hexanoyl]amino]-3-phenylpropanoyl]amino]hexanoic acid;2,2,2-trifluoroacetic acid |
Source
|
---|---|---|
Details | Computed by LexiChem 2.6.6 (PubChem release 2019.06.18) | |
Source | PubChem | |
URL | https://pubchem.ncbi.nlm.nih.gov | |
Description | Data deposited in or computed by PubChem | |
InChI |
InChI=1S/C55H96N14O13.C2HF3O2/c1-5-33(3)45(69-51(77)40(25-27-44(71)72)65-54(80)46(34(4)6-2)68-50(76)39(24-26-43(61)70)62-47(73)36(60)20-10-14-28-56)53(79)64-38(22-12-16-30-58)48(74)63-37(21-11-15-29-57)49(75)67-42(32-35-18-8-7-9-19-35)52(78)66-41(55(81)82)23-13-17-31-59;3-2(4,5)1(6)7/h7-9,18-19,33-34,36-42,45-46H,5-6,10-17,20-32,56-60H2,1-4H3,(H2,61,70)(H,62,73)(H,63,74)(H,64,79)(H,65,80)(H,66,78)(H,67,75)(H,68,76)(H,69,77)(H,71,72)(H,81,82);(H,6,7)/t33-,34-,36-,37-,38-,39-,40-,41-,42-,45-,46-;/m0./s1 |
Source
|
Details | Computed by InChI 1.0.5 (PubChem release 2019.06.18) | |
Source | PubChem | |
URL | https://pubchem.ncbi.nlm.nih.gov | |
Description | Data deposited in or computed by PubChem | |
InChI Key |
UBIXVFFLYALTSN-TWIANDLUSA-N |
Source
|
Details | Computed by InChI 1.0.5 (PubChem release 2019.06.18) | |
Source | PubChem | |
URL | https://pubchem.ncbi.nlm.nih.gov | |
Description | Data deposited in or computed by PubChem | |
Canonical SMILES |
CCC(C)C(C(=O)NC(CCC(=O)O)C(=O)NC(C(C)CC)C(=O)NC(CCCCN)C(=O)NC(CCCCN)C(=O)NC(CC1=CC=CC=C1)C(=O)NC(CCCCN)C(=O)O)NC(=O)C(CCC(=O)N)NC(=O)C(CCCCN)N.C(=O)(C(F)(F)F)O |
Source
|
Details | Computed by OEChem 2.1.5 (PubChem release 2019.06.18) | |
Source | PubChem | |
URL | https://pubchem.ncbi.nlm.nih.gov | |
Description | Data deposited in or computed by PubChem | |
Isomeric SMILES |
CC[C@H](C)[C@@H](C(=O)N[C@@H](CCC(=O)O)C(=O)N[C@@H]([C@@H](C)CC)C(=O)N[C@@H](CCCCN)C(=O)N[C@@H](CCCCN)C(=O)N[C@@H](CC1=CC=CC=C1)C(=O)N[C@@H](CCCCN)C(=O)O)NC(=O)[C@H](CCC(=O)N)NC(=O)[C@H](CCCCN)N.C(=O)(C(F)(F)F)O |
Source
|
Details | Computed by OEChem 2.1.5 (PubChem release 2019.06.18) | |
Source | PubChem | |
URL | https://pubchem.ncbi.nlm.nih.gov | |
Description | Data deposited in or computed by PubChem | |
Molecular Formula |
C57H97F3N14O15 |
Source
|
Details | Computed by PubChem 2.1 (PubChem release 2019.06.18) | |
Source | PubChem | |
URL | https://pubchem.ncbi.nlm.nih.gov | |
Description | Data deposited in or computed by PubChem | |
Molecular Weight |
1275.5 g/mol |
Source
|
Details | Computed by PubChem 2.1 (PubChem release 2021.05.07) | |
Source | PubChem | |
URL | https://pubchem.ncbi.nlm.nih.gov | |
Description | Data deposited in or computed by PubChem | |
Disclaimer and Information on In-Vitro Research Products
Please be aware that all articles and product information presented on BenchChem are intended solely for informational purposes. The products available for purchase on BenchChem are specifically designed for in-vitro studies, which are conducted outside of living organisms. In-vitro studies, derived from the Latin term "in glass," involve experiments performed in controlled laboratory settings using cells or tissues. It is important to note that these products are not categorized as medicines or drugs, and they have not received approval from the FDA for the prevention, treatment, or cure of any medical condition, ailment, or disease. We must emphasize that any form of bodily introduction of these products into humans or animals is strictly prohibited by law. It is essential to adhere to these guidelines to ensure compliance with legal and ethical standards in research and experimentation.