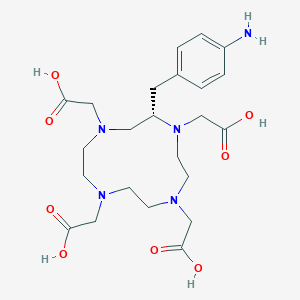
para-Aminobenzyl-DOTA
- Click on QUICK INQUIRY to receive a quote from our team of experts.
- With the quality product at a COMPETITIVE price, you can focus more on your research.
Overview
Description
para-Aminobenzyl-DOTA is a macrocyclic compound known for its ability to form stable complexes with metal ions. This compound is particularly significant in the field of biomedical imaging and radiopharmaceuticals due to its high affinity for metal ions and its ability to form stable chelates .
Preparation Methods
Synthetic Routes and Reaction Conditions
The reaction conditions often require the use of strong bases and high temperatures to facilitate the cyclization process .
Industrial Production Methods
Industrial production of this compound involves large-scale synthesis using automated reactors to ensure consistency and purity. The process includes multiple purification steps such as crystallization and chromatography to achieve the desired product quality .
Chemical Reactions Analysis
Types of Reactions
para-Aminobenzyl-DOTA undergoes various chemical reactions including:
Complexation: Forms stable complexes with metal ions such as gadolinium, copper, and gallium.
Substitution: The amino group can participate in substitution reactions to form derivatives with different functional groups.
Common Reagents and Conditions
Complexation: Metal salts (e.g., gadolinium chloride) in aqueous solutions under mild conditions.
Substitution: Reagents such as acyl chlorides or sulfonyl chlorides in the presence of a base.
Major Products
Scientific Research Applications
para-Aminobenzyl-DOTA has a wide range of applications in scientific research:
Mechanism of Action
The compound exerts its effects primarily through its ability to form stable complexes with metal ions. The macrocyclic structure provides a rigid framework that encapsulates the metal ion, preventing its release and ensuring stability. This property is crucial for its use in imaging and therapeutic applications where stability and specificity are paramount .
Comparison with Similar Compounds
Similar Compounds
1,4,7,10-Tetraazacyclododecane-1,4,7,10-tetraacetic acid: Similar in structure but lacks the 2-[(4-aminophenyl)methyl] group.
1,4,8,11-Tetraazacyclotetradecane: Another macrocyclic compound with a different ring size and coordination properties.
Diethylenetriaminepentaacetic acid: A linear polyamine with multiple acetic acid groups, used for similar applications but with different complexation properties.
Uniqueness
para-Aminobenzyl-DOTA is unique due to its specific functionalization, which allows for targeted applications in imaging and therapy. The presence of the 2-[(4-aminophenyl)methyl] group provides additional sites for conjugation with biomolecules, enhancing its versatility and effectiveness in various applications .
Biological Activity
Introduction
Para-Aminobenzyl-DOTA (p-AB-DOTA) is a derivative of the chelating agent DOTA (1,4,7,10-tetraazacyclododecane-1,4,7,10-tetraacetic acid) that has gained attention in the field of radiopharmaceuticals and targeted therapy. Its unique structure allows for enhanced binding to metal ions, making it particularly useful in the development of radiolabeled compounds for imaging and therapeutic applications in oncology. This article reviews the biological activity of p-AB-DOTA, focusing on its pharmacokinetics, biodistribution, and therapeutic efficacy based on recent studies.
Chemical Structure and Properties
The chemical structure of this compound incorporates an amino group that enhances its functionality for various biomedical applications. The presence of the para-aminobenzyl group improves solubility and facilitates conjugation with biomolecules such as antibodies or peptides.
Pharmacokinetics
Pharmacokinetic studies have shown that p-AB-DOTA exhibits rapid clearance from the bloodstream and low retention in normal tissues. A study evaluating the biodistribution of 177Lu-labeled p-AB-DOTA in CD1 mice reported that only 2–4% of the injected dose remained in the body four hours post-injection. The kidneys were identified as the dose-limiting organ due to their significant uptake of the radiolabeled compound .
Table 1: Pharmacokinetic Parameters of p-AB-DOTA
Parameter | Value |
---|---|
Blood Clearance Half-life | 2 hours |
Whole-body Retention | <5% at 4 hours post-injection |
Estimated Kidney Dose | 0.1 mGy/MBq |
Biodistribution Studies
Biodistribution studies have demonstrated that p-AB-DOTA derivatives exhibit favorable tumor uptake characteristics. For instance, a comparative analysis of various DOTA-based haptens indicated that increasing binding affinity correlates with enhanced tumor accumulation. Specifically, 177Lu-DOTA showed a tumor activity of 14.3 ± 1.8 %ID/g in tumor-bearing mice, significantly higher than lower-affinity variants .
Table 2: Tumor Uptake of DOTA Derivatives
Compound | Tumor Uptake (%ID/g) | Binding Affinity (K_D) |
---|---|---|
111In-DOTA | 0.5 ± 0.1 | 20 nM |
111In-DOTABn | 1.6 ± 0.3 | 1 nM |
177Lu-DOTA | 14.3 ± 1.8 | 400 pM |
177Lu-DOTABn | 19 ± 4 | 10 pM |
Therapeutic Applications
The therapeutic potential of p-AB-DOTA is being explored in targeted radionuclide therapy (TRT). One notable application is its use in peptide receptor radionuclide therapy (PRRT) for neuroendocrine tumors. Clinical studies have shown promising results using radiolabeled somatostatin analogs containing p-AB-DOTA, demonstrating significant tumor regression and manageable side effects .
Case Studies
In a clinical setting, a study involving patients with metastatic neuroendocrine tumors treated with ^177Lu-DOTATATE (a DOTA-based compound) reported an overall response rate of approximately 30%. Patients exhibited improved quality of life and symptom relief with manageable toxicity profiles .
Another preclinical study assessed the efficacy of a bispecific antibody conjugated to p-AB-DOTA for targeted therapy against GD2-positive tumors. The results indicated enhanced tumor targeting and reduced off-target effects compared to conventional therapies .
This compound represents a significant advancement in the development of targeted radiopharmaceuticals due to its favorable pharmacokinetic profile and high tumor uptake capabilities. Ongoing research continues to explore its full potential in various therapeutic applications, particularly in oncology. Future studies will likely focus on optimizing its binding properties and expanding its use across different cancer types.
Properties
IUPAC Name |
2-[(6S)-6-[(4-aminophenyl)methyl]-4,7,10-tris(carboxymethyl)-1,4,7,10-tetrazacyclododec-1-yl]acetic acid |
Source
|
---|---|---|
Details | Computed by LexiChem 2.6.6 (PubChem release 2019.06.18) | |
Source | PubChem | |
URL | https://pubchem.ncbi.nlm.nih.gov | |
Description | Data deposited in or computed by PubChem | |
InChI |
InChI=1S/C23H35N5O8/c24-18-3-1-17(2-4-18)11-19-12-27(15-22(33)34)8-7-25(13-20(29)30)5-6-26(14-21(31)32)9-10-28(19)16-23(35)36/h1-4,19H,5-16,24H2,(H,29,30)(H,31,32)(H,33,34)(H,35,36)/t19-/m0/s1 |
Source
|
Details | Computed by InChI 1.0.5 (PubChem release 2019.06.18) | |
Source | PubChem | |
URL | https://pubchem.ncbi.nlm.nih.gov | |
Description | Data deposited in or computed by PubChem | |
InChI Key |
VEYDUAGNNSZVTN-IBGZPJMESA-N |
Source
|
Details | Computed by InChI 1.0.5 (PubChem release 2019.06.18) | |
Source | PubChem | |
URL | https://pubchem.ncbi.nlm.nih.gov | |
Description | Data deposited in or computed by PubChem | |
Canonical SMILES |
C1CN(CCN(C(CN(CCN1CC(=O)O)CC(=O)O)CC2=CC=C(C=C2)N)CC(=O)O)CC(=O)O |
Source
|
Details | Computed by OEChem 2.1.5 (PubChem release 2019.06.18) | |
Source | PubChem | |
URL | https://pubchem.ncbi.nlm.nih.gov | |
Description | Data deposited in or computed by PubChem | |
Isomeric SMILES |
C1CN(CCN([C@H](CN(CCN1CC(=O)O)CC(=O)O)CC2=CC=C(C=C2)N)CC(=O)O)CC(=O)O |
Source
|
Details | Computed by OEChem 2.1.5 (PubChem release 2019.06.18) | |
Source | PubChem | |
URL | https://pubchem.ncbi.nlm.nih.gov | |
Description | Data deposited in or computed by PubChem | |
Molecular Formula |
C23H35N5O8 |
Source
|
Details | Computed by PubChem 2.1 (PubChem release 2019.06.18) | |
Source | PubChem | |
URL | https://pubchem.ncbi.nlm.nih.gov | |
Description | Data deposited in or computed by PubChem | |
Molecular Weight |
509.6 g/mol |
Source
|
Details | Computed by PubChem 2.1 (PubChem release 2021.05.07) | |
Source | PubChem | |
URL | https://pubchem.ncbi.nlm.nih.gov | |
Description | Data deposited in or computed by PubChem | |
Disclaimer and Information on In-Vitro Research Products
Please be aware that all articles and product information presented on BenchChem are intended solely for informational purposes. The products available for purchase on BenchChem are specifically designed for in-vitro studies, which are conducted outside of living organisms. In-vitro studies, derived from the Latin term "in glass," involve experiments performed in controlled laboratory settings using cells or tissues. It is important to note that these products are not categorized as medicines or drugs, and they have not received approval from the FDA for the prevention, treatment, or cure of any medical condition, ailment, or disease. We must emphasize that any form of bodily introduction of these products into humans or animals is strictly prohibited by law. It is essential to adhere to these guidelines to ensure compliance with legal and ethical standards in research and experimentation.