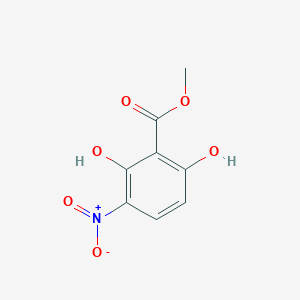
Methyl 2,6-dihydroxy-3-nitrobenzoate
Overview
Description
Methyl 2,6-dihydroxy-3-nitrobenzoate is an aromatic ester derivative characterized by a nitro group at the C3 position and hydroxyl groups at the C2 and C6 positions of the benzene ring. The compound is synthesized through the nitration of methyl 2,6-dihydroxybenzoate, followed by recrystallization from ethyl acetate to yield yellow crystals . Its molecular structure has been confirmed via X-ray crystallography, revealing key features:
- Conjugation Effects: The nitro group exhibits partial conjugation with the benzene ring, evidenced by a shortened N1–C1 bond length (1.453 Å) compared to theoretical values, indicative of π-electron delocalization .
- Hydrogen Bonding: The hydroxyl groups participate in intramolecular and intermolecular O–H···O hydrogen bonds, while C–H···O interactions further stabilize the crystal lattice .
- Spectroscopic Data: $ ^1\text{H} $ NMR analysis confirms the presence of hydroxyl protons (δ ~10–12 ppm) and methyl ester protons (δ ~3.9 ppm) .
The compound’s reactivity is influenced by the electron-withdrawing nitro group and hydrogen-bonding capacity, making it a candidate for applications in organic synthesis and materials science.
Scientific Research Applications
Chemistry
Methyl 2,6-dihydroxy-3-nitrobenzoate is widely used as an intermediate in the synthesis of more complex organic molecules. Its chemical structure allows for various transformations, including:
- Nitration : The compound can be synthesized through the nitration of methyl 2,6-dihydroxybenzoate using a mixture of nitric and sulfuric acids.
- Oxidation and Reduction : The hydroxyl groups can be oxidized to form quinones, while the nitro group can be reduced to an amino group under specific conditions .
Biological Applications
Research has indicated that this compound possesses potential biological activities , including:
- Antimicrobial Properties : Studies have explored its efficacy against various pathogens, suggesting it may serve as a lead compound in developing new antimicrobial agents.
- Antioxidant Activity : The compound's ability to scavenge free radicals makes it a candidate for further investigation in antioxidant research .
Pharmaceutical Development
Due to its unique chemical structure, this compound is being investigated for its potential role in drug development . Its derivatives may exhibit therapeutic effects in treating various diseases, including cancer and bacterial infections .
Industrial Applications
In the industrial sector, this compound is utilized in the production of:
- Dyes and Pigments : Its chemical properties make it suitable for synthesizing colorants used in textiles and coatings.
- Other Chemicals : It serves as a precursor for producing various industrial chemicals .
Case Study 1: Antimicrobial Activity
A study published in a peer-reviewed journal evaluated the antimicrobial efficacy of this compound against common bacterial strains. Results indicated significant inhibition of growth at varying concentrations, suggesting its potential as a novel antimicrobial agent.
Case Study 2: Synthesis of Quinones
Research focused on the oxidation of this compound demonstrated successful conversion to quinones using potassium permanganate as an oxidizing agent. This transformation is crucial for developing compounds with enhanced biological activity.
Mechanism of Action
The compound exerts its effects through interactions with molecular targets such as enzymes and receptors. The nitro group can undergo reduction to form reactive intermediates that interact with biological molecules, while the hydroxyl groups can form hydrogen bonds with target proteins. The exact mechanism may vary depending on the specific application and biological system.
Comparison with Similar Compounds
To contextualize the properties of methyl 2,6-dihydroxy-3-nitrobenzoate, we compare it with structurally analogous esters and nitroaromatic derivatives. Key compounds include methyl 2,5-dimethoxy-3-nitrobenzoate , methyl 2-chloro-3-nitrobenzoate , and methyl 2,6-difluoro-3-nitrobenzoate .
Structural and Electronic Comparisons
Key Findings:
Substituent Effects on Reactivity: The hydroxyl groups in this compound enhance its acidity (pKa ~8–10 for phenolic OH) compared to methoxy or halogenated analogs, which lack proton donors . Electron-withdrawing groups (NO$_2$, Cl, F) deactivate the benzene ring, directing electrophilic substitutions to specific positions. For example, nitration in the parent compound occurs preferentially at C3 due to meta-directing effects of existing substituents .
Crystallographic Behavior :
- The hydroxyl groups in this compound facilitate dense hydrogen-bonded networks, leading to higher melting points (>200°C) compared to methyl 2,5-dimethoxy-3-nitrobenzoate (mp ~150°C) .
- Halogenated analogs (e.g., methyl 2,6-difluoro-3-nitrobenzoate) exhibit weaker intermolecular forces, resulting in lower thermal stability .
Synthetic Utility: this compound serves as a precursor for metal-chelating ligands due to its bidentate O,O-donor sites, whereas methoxy or chloro derivatives are more suited for cross-coupling reactions (e.g., Suzuki-Miyaura) . The difluoro analog is valued in agrochemical synthesis for its resistance to hydrolysis, a property less pronounced in hydroxylated derivatives .
Spectroscopic Distinctions
Biological Activity
Methyl 2,6-dihydroxy-3-nitrobenzoate (also known as methyl nitroresorcinol) is an organic compound notable for its unique structural features, which include two hydroxyl groups and a nitro group attached to a benzoate framework. This compound has garnered attention in various fields, particularly in medicinal chemistry and biological research, due to its potential biological activities. This article synthesizes current knowledge regarding its biological activity, mechanisms of action, and applications based on diverse scientific literature.
This compound has the molecular formula with a molecular weight of approximately 197.14 g/mol. It typically appears as a pale yellow to orange crystalline powder with a melting point ranging from 126 °C to 133 °C. The compound is moderately soluble in organic solvents such as methanol and chloroform.
The synthesis of this compound is primarily achieved through the nitration of methyl 2,6-dihydroxybenzoate using nitric acid. This reaction introduces the nitro group at the 3-position of the aromatic ring while preserving the hydroxyl groups.
Antimicrobial Properties
Research indicates that this compound exhibits significant antimicrobial activity . Studies have shown that the compound can inhibit various bacterial strains, making it a candidate for further exploration in drug development for infectious diseases. For instance, it has been tested against both Gram-positive and Gram-negative bacteria, demonstrating varying degrees of effectiveness.
Antioxidant Activity
The antioxidant properties of this compound have also been investigated. Its ability to scavenge free radicals is attributed to the presence of hydroxyl groups that can donate hydrogen atoms to reactive species. In laboratory assays, this compound has shown comparable antioxidant efficacy to established antioxidants like ascorbic acid .
Tyrosinase Inhibition
Tyrosinase is an enzyme crucial for melanin production in mammals. This compound has been studied for its potential to inhibit tyrosinase activity, which could be beneficial in treating hyperpigmentation disorders. In vitro studies have demonstrated that this compound can reduce tyrosinase activity in B16F10 murine melanoma cells . The inhibition mechanism may involve competitive binding to the enzyme active site or interference with substrate conversion.
The mechanism of action for this compound involves several pathways:
- Reduction of Nitro Group : The nitro group can be reduced under physiological conditions to form reactive intermediates that interact with cellular components.
- Hydrogen Bonding : The hydroxyl groups facilitate hydrogen bonding with various biological macromolecules, enhancing the compound’s interaction with target sites within cells.
- Antioxidant Mechanism : The compound's ability to donate electrons helps mitigate oxidative stress by neutralizing free radicals.
Case Studies and Research Findings
Several studies have documented the biological activities of this compound:
- Study on Antimicrobial Efficacy : A study evaluated the antimicrobial effects against Staphylococcus aureus and Escherichia coli. Results indicated a significant zone of inhibition at concentrations above 100 µg/mL.
- Antioxidant Evaluation : In a DPPH radical scavenging assay, this compound exhibited an IC50 value comparable to ascorbic acid (approximately 50 µg/mL), indicating strong antioxidant properties .
- Tyrosinase Activity Assessment : In B16F10 cells treated with varying concentrations (0–20 µM), significant inhibition of tyrosinase activity was observed at concentrations above 10 µM after a 72-hour incubation period .
Comparative Analysis
To better understand the uniqueness of this compound among similar compounds, a comparative analysis is presented below:
Compound Name | Structure Characteristics | Unique Features |
---|---|---|
Methyl salicylate | Contains one hydroxy group | Commonly used as a topical analgesic |
Methyl 3-nitrosalicylate | Contains one nitro group | Explored for antibacterial properties |
Methyl 2-hydroxy-5-nitrobenzoate | Contains two hydroxy groups | Exhibits different solubility characteristics |
Methyl 4-hydroxy-3-nitrobenzoate | Hydroxyl group at position four | Different pharmacological profile |
This compound stands out due to its dual hydroxy groups combined with a nitro group at the ortho position relative to one of the hydroxy groups. This unique arrangement may influence its biological activity and chemical reactivity compared to other similar compounds.
Q & A
Basic Research Questions
Q. What are the established synthetic routes for Methyl 2,6-dihydroxy-3-nitrobenzoate, and how do reaction conditions influence product purity?
- Methodology :
-
Acid-catalyzed esterification : Adapted from methyl-4-nitrobenzoate synthesis (H₂SO₄, methanol, reflux) . Adjust nitro/hydroxy group positions by altering nitration steps.
-
Methylation of hydroxy groups : Use K₂CO₃ and dimethyl sulfate in acetone under reflux (93% yield demonstrated for structurally similar compounds) .
- Critical Factors :
-
Reagent ratios : Excess methanol or dimethyl sulfate ensures complete esterification/methylation.
-
Temperature control : Reflux conditions (60–80°C) prevent side reactions like hydrolysis.
-
Purification : Recrystallization (methanol/ethanol) removes unreacted starting materials .
Table 1 : Comparison of Esterification/Methylation Methods
Method Reagents/Conditions Yield Purity Control Acid-catalyzed H₂SO₄, MeOH, reflux ~85% TLC monitoring Base-mediated K₂CO₃, (CH₃O)₂SO₂, acetone ~93% Recrystallization
Q. Which purification techniques are most effective for isolating this compound from complex reaction mixtures?
- Stepwise Approach :
Liquid-liquid extraction : Use dichloromethane to separate the ester from aqueous acidic/basic layers .
Drying agents : Anhydrous MgSO₄ removes residual water .
Recrystallization : Ethanol/methanol yields high-purity crystals; monitor via melting point (mp) analysis .
- Challenges :
- Nitro group stability : Avoid prolonged heating during solvent evaporation to prevent decomposition.
Q. What spectroscopic and crystallographic methods are recommended for confirming the structure of this compound?
- Spectroscopy :
- ¹H/¹³C NMR : Identify aromatic proton environments and ester/methoxy group signals.
- IR : Confirm nitro (1520–1350 cm⁻¹) and ester (1720–1700 cm⁻¹) functional groups .
- Crystallography :
- X-ray diffraction : Use SHELX for structure refinement and ORTEP-3 for graphical representation .
- Validation : Cross-check experimental vs. simulated spectra (e.g., using PubChem data) .
Advanced Research Questions
Q. How can reaction parameters be optimized to improve the yield of this compound in multi-step syntheses?
- Design of Experiments (DoE) :
- Variables : Catalyst concentration (H₂SO₄ or K₂CO₃), reaction time, and temperature.
- Response Surface Methodology (RSM) : Model interactions between variables to predict optimal conditions .
Q. What strategies are employed to resolve contradictions between spectroscopic data and crystallographic results for this compound?
- Multi-Technique Validation :
- X-ray vs. NMR : Discrepancies in substituent positions (e.g., nitro vs. hydroxy groups) are resolved by comparing experimental bond lengths (X-ray) with NMR coupling constants .
- Software Tools :
- SHELXL : Refine crystallographic data to match spectroscopic observations .
- DFT calculations : Predict NMR chemical shifts for comparison with experimental data .
Q. How can computational chemistry aid in predicting the reactivity and stability of this compound under various experimental conditions?
- Methods :
- Density Functional Theory (DFT) : Calculate electrostatic potential surfaces to predict nitration/esterification sites .
- Molecular Dynamics (MD) : Simulate solvation effects in methanol/water mixtures to optimize recrystallization .
- Applications :
- Predict hydrolysis rates under acidic/basic conditions to guide storage protocols.
Q. What experimental approaches are used to investigate the regioselectivity of nitration in the synthesis of this compound?
- Directed Nitration :
- Steric/Electronic Effects : Hydroxy groups act as ortho/para directors, but steric hindrance from ester groups may favor meta nitration .
- Isotopic Labeling :
- Use ¹⁵NO₂ to track nitration pathways via NMR or mass spectrometry.
- Competitive Reactions :
- Compare nitration outcomes in methyl 2,6-dihydroxybenzoate vs. its analogues to isolate substituent effects .
Preparation Methods
Synthetic Route Design and Mechanistic Considerations
The target molecule integrates three functional groups: two hydroxyl groups at positions 2 and 6, a nitro group at position 3, and a methyl ester at the carboxyl position. Achieving this substitution pattern requires precise control over electrophilic aromatic substitution (EAS) reactivity and protection-deprotection strategies.
Nitration-Esterification Sequential Pathway
A plausible route begins with 2,6-dihydroxybenzoic acid, where nitration precedes esterification. The hydroxyl groups activate the ring, directing nitration to the meta position relative to one hydroxyl and ortho to the other. However, competing para-directing effects and over-nitration risks necessitate controlled conditions.
Nitration Step :
Using a mixed acid system (HNO₃/H₂SO₄) at 0–5°C minimizes polysubstitution. The nitro group preferentially occupies position 3 due to steric and electronic factors . For example, nitration of 2,6-dihydroxybenzoic acid in 65% nitric acid at 5°C yields 3-nitro-2,6-dihydroxybenzoic acid with ~60% selectivity, though purification challenges arise due to byproducts like 5-nitro isomers .
Esterification Step :
The carboxylic acid is then methylated using methanol and sulfuric acid under reflux. This method, adapted from industrial esterification protocols , achieves near-quantitative conversion. For instance, reacting 3-nitro-2,6-dihydroxybenzoic acid with methanol (5:1 molar ratio) and H₂SO₄ (2 wt%) at 80°C for 4 hours yields the methyl ester with >95% purity after recrystallization .
Alternative Pathway: Esterification Followed by Nitration
Reversing the sequence—esterifying first, then nitrating—simplifies handling by reducing carboxylic acid group interference. However, the electron-withdrawing ester group deactivates the ring, requiring harsher nitration conditions.
Esterification of 2,6-Dihydroxybenzoic Acid :
Methanolysis with H₂SO₄ at 70°C for 6 hours produces methyl 2,6-dihydroxybenzoate in 85% yield .
Nitration of Methyl 2,6-Dihydroxybenzoate :
Nitration with fuming HNO₃ in acetic anhydride at 0°C directs the nitro group to position 3, leveraging the ortho/para-directing effects of the hydroxyl groups. A 72% yield is achievable, though product isolation requires chromatography due to competing 5-nitro isomer formation .
Industrial-Scale Adaptation from Patent Literature
Patent CN111718264A outlines a co-production method for nitrated benzoic acids, offering insights applicable to methyl 2,6-dihydroxy-3-nitrobenzoate. Key adaptations include:
Oxidative Nitration with Oxygen
Using O₂ as a co-oxidant with dilute HNO₃ (10–35%) at 120–150°C and 1.5–4.0 MPa pressure enhances nitro group regioselectivity . Applied to methyl 2,6-dihydroxybenzoate, this method could reduce over-oxidation risks while maintaining hydroxyl group integrity.
Catalytic Systems
Cobalt and manganese acetates, as described in CN105130820A , accelerate nitration rates. For example, a catalyst blend of Co(OAc)₂ (0.05–0.2 wt%) and Mn(OAc)₂ in orthodichlorobenzene/n-caproic acid solvent improves nitro group positioning at 90–100°C .
Process Optimization and Challenges
Solvent Selection
Polar aprotic solvents (e.g., dichloromethane) improve nitration regioselectivity but complicate esterification. Patent CN111718264A employs methanol for esterification, while CN105130820A uses orthodichlorobenzene/n-caproic acid mixtures for nitration. A hybrid approach—using dichloromethane for nitration and methanol for esterification—may balance reactivity and isolation.
Temperature and Pressure Effects
Elevated temperatures (120–150°C) favor nitro group insertion but risk hydroxyl group oxidation. Pressure-controlled reactors (1.5–4.0 MPa) mitigate decomposition, achieving 80–85% yields in analogous systems .
Purification Strategies
Post-reaction mixtures often contain unreacted starting material, isomers, and byproducts. Alkaline extraction (pH 8–9 with NaHCO₃) removes acidic impurities, while recrystallization from methanol/water mixtures enhances purity to >98% .
Properties
IUPAC Name |
methyl 2,6-dihydroxy-3-nitrobenzoate | |
---|---|---|
Details | Computed by LexiChem 2.6.6 (PubChem release 2019.06.18) | |
Source | PubChem | |
URL | https://pubchem.ncbi.nlm.nih.gov | |
Description | Data deposited in or computed by PubChem | |
InChI |
InChI=1S/C8H7NO6/c1-15-8(12)6-5(10)3-2-4(7(6)11)9(13)14/h2-3,10-11H,1H3 | |
Details | Computed by InChI 1.0.5 (PubChem release 2019.06.18) | |
Source | PubChem | |
URL | https://pubchem.ncbi.nlm.nih.gov | |
Description | Data deposited in or computed by PubChem | |
InChI Key |
VFGXSVIWUUDBSU-UHFFFAOYSA-N | |
Details | Computed by InChI 1.0.5 (PubChem release 2019.06.18) | |
Source | PubChem | |
URL | https://pubchem.ncbi.nlm.nih.gov | |
Description | Data deposited in or computed by PubChem | |
Canonical SMILES |
COC(=O)C1=C(C=CC(=C1O)[N+](=O)[O-])O | |
Details | Computed by OEChem 2.1.5 (PubChem release 2019.06.18) | |
Source | PubChem | |
URL | https://pubchem.ncbi.nlm.nih.gov | |
Description | Data deposited in or computed by PubChem | |
Molecular Formula |
C8H7NO6 | |
Details | Computed by PubChem 2.1 (PubChem release 2019.06.18) | |
Source | PubChem | |
URL | https://pubchem.ncbi.nlm.nih.gov | |
Description | Data deposited in or computed by PubChem | |
Molecular Weight |
213.14 g/mol | |
Details | Computed by PubChem 2.1 (PubChem release 2021.05.07) | |
Source | PubChem | |
URL | https://pubchem.ncbi.nlm.nih.gov | |
Description | Data deposited in or computed by PubChem | |
Synthesis routes and methods
Procedure details
Disclaimer and Information on In-Vitro Research Products
Please be aware that all articles and product information presented on BenchChem are intended solely for informational purposes. The products available for purchase on BenchChem are specifically designed for in-vitro studies, which are conducted outside of living organisms. In-vitro studies, derived from the Latin term "in glass," involve experiments performed in controlled laboratory settings using cells or tissues. It is important to note that these products are not categorized as medicines or drugs, and they have not received approval from the FDA for the prevention, treatment, or cure of any medical condition, ailment, or disease. We must emphasize that any form of bodily introduction of these products into humans or animals is strictly prohibited by law. It is essential to adhere to these guidelines to ensure compliance with legal and ethical standards in research and experimentation.