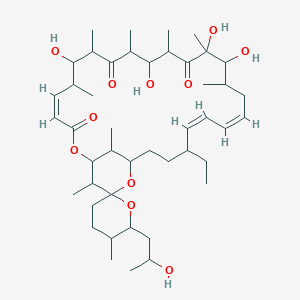
Oligomycin
- Click on QUICK INQUIRY to receive a quote from our team of experts.
- With the quality product at a COMPETITIVE price, you can focus more on your research.
Overview
Description
The compound (4Z,18Z,20Z)-22-ethyl-7,11,14,15-tetrahydroxy-6’-(2-hydroxypropyl)-5’,6,8,10,12,14,16,28,29-nonamethylspiro[2,26-dioxabicyclo[2331]nonacosa-4,18,20-triene-27,2’-oxane]-3,9,13-trione is a complex organic molecule with a unique structure It is characterized by multiple hydroxyl groups, a spiro linkage, and a nonacosa-triene backbone
Preparation Methods
Synthetic Routes and Reaction Conditions
The synthesis of this compound involves multiple steps, starting from simpler organic molecules. The key steps include:
- Formation of the nonacosa-triene backbone through a series of aldol condensations and cyclization reactions.
- Introduction of the spiro linkage via a spirocyclization reaction.
- Functionalization of the molecule with hydroxyl groups through selective hydroxylation reactions.
- Final steps involve purification and characterization of the compound using techniques such as chromatography and spectroscopy.
Industrial Production Methods
Industrial production of this compound would require optimization of the synthetic route to ensure high yield and purity. This could involve the use of advanced catalytic systems and continuous flow reactors to streamline the synthesis process. Additionally, large-scale purification techniques such as crystallization and distillation would be employed to obtain the final product.
Chemical Reactions Analysis
Types of Reactions
This compound can undergo various chemical reactions, including:
Oxidation: The hydroxyl groups can be oxidized to form ketones or aldehydes.
Reduction: The compound can be reduced to form alcohols or alkanes.
Substitution: The hydroxyl groups can be substituted with other functional groups such as halides or amines.
Common Reagents and Conditions
Oxidation: Reagents such as potassium permanganate or chromium trioxide can be used under acidic or basic conditions.
Reduction: Reagents such as lithium aluminum hydride or sodium borohydride can be used under anhydrous conditions.
Substitution: Reagents such as thionyl chloride or phosphorus tribromide can be used for halogenation reactions.
Major Products
The major products formed from these reactions include:
Oxidation: Ketones, aldehydes, or carboxylic acids.
Reduction: Alcohols or alkanes.
Substitution: Halides, amines, or ethers.
Scientific Research Applications
Chemistry: It can be used as a building block for the synthesis of more complex molecules.
Biology: It can be studied for its potential biological activity, including its effects on cellular processes.
Medicine: It may have potential as a therapeutic agent for the treatment of various diseases.
Industry: It can be used in the development of new materials with unique properties.
Mechanism of Action
The mechanism of action of this compound involves its interaction with specific molecular targets. These targets may include enzymes, receptors, or other proteins involved in cellular processes. The compound may exert its effects by binding to these targets and modulating their activity, leading to changes in cellular function.
Comparison with Similar Compounds
Similar Compounds
- (4E,18Z,20Z)-22-ethyl-7,11,14,15,28-pentahydroxy-6’-(2-hydroxypropyl)-5’,6,8,10,12,14,16,28,29-nonamethyl-2,26-dioxaspiro[bicyclo[23.3.1]nonacosane-27,2’-oxane]-4,18,20-triene-3,3’,9,13-tetrone .
- 22-Ethyl-7,9,11,15-tetrahydroxy-6’-(2-hydroxypropyl)-5’,6,8,10,14,16,28,29-octamethylspiro[2,26-dioxabicyclo[23.3.1]nonacosa-4,18,20-triene-27,2’-oxane]-3,13-dione .
Uniqueness
The uniqueness of (4Z,18Z,20Z)-22-ethyl-7,11,14,15-tetrahydroxy-6’-(2-hydroxypropyl)-5’,6,8,10,12,14,16,28,29-nonamethylspiro[2,26-dioxabicyclo[23.3.1]nonacosa-4,18,20-triene-27,2’-oxane]-3,9,13-trione lies in its specific arrangement of functional groups and its spiro linkage, which confer unique chemical and biological properties. This makes it a valuable compound for further research and development in various fields.
Properties
IUPAC Name |
(4Z,18Z,20Z)-22-ethyl-7,11,14,15-tetrahydroxy-6'-(2-hydroxypropyl)-5',6,8,10,12,14,16,28,29-nonamethylspiro[2,26-dioxabicyclo[23.3.1]nonacosa-4,18,20-triene-27,2'-oxane]-3,9,13-trione |
Source
|
---|---|---|
Details | Computed by LexiChem 2.6.6 (PubChem release 2019.06.18) | |
Source | PubChem | |
URL | https://pubchem.ncbi.nlm.nih.gov | |
Description | Data deposited in or computed by PubChem | |
InChI |
InChI=1S/C45H74O11/c1-12-34-17-15-13-14-16-27(4)42(51)44(11,53)43(52)32(9)40(50)31(8)39(49)30(7)38(48)26(3)18-21-37(47)54-41-29(6)35(20-19-34)55-45(33(41)10)23-22-25(2)36(56-45)24-28(5)46/h13-15,17-18,21,25-36,38,40-42,46,48,50-51,53H,12,16,19-20,22-24H2,1-11H3/b14-13-,17-15-,21-18- |
Source
|
Details | Computed by InChI 1.0.5 (PubChem release 2019.06.18) | |
Source | PubChem | |
URL | https://pubchem.ncbi.nlm.nih.gov | |
Description | Data deposited in or computed by PubChem | |
InChI Key |
MNULEGDCPYONBU-YNZHUHFTSA-N |
Source
|
Details | Computed by InChI 1.0.5 (PubChem release 2019.06.18) | |
Source | PubChem | |
URL | https://pubchem.ncbi.nlm.nih.gov | |
Description | Data deposited in or computed by PubChem | |
Canonical SMILES |
CCC1CCC2C(C(C(C3(O2)CCC(C(O3)CC(C)O)C)C)OC(=O)C=CC(C(C(C(=O)C(C(C(C(=O)C(C(C(CC=CC=C1)C)O)(C)O)C)O)C)C)O)C)C |
Source
|
Details | Computed by OEChem 2.1.5 (PubChem release 2019.06.18) | |
Source | PubChem | |
URL | https://pubchem.ncbi.nlm.nih.gov | |
Description | Data deposited in or computed by PubChem | |
Isomeric SMILES |
CCC\1CCC2C(C(C(C3(O2)CCC(C(O3)CC(C)O)C)C)OC(=O)/C=C\C(C(C(C(=O)C(C(C(C(=O)C(C(C(C/C=C\C=C1)C)O)(C)O)C)O)C)C)O)C)C |
Source
|
Details | Computed by OEChem 2.1.5 (PubChem release 2019.06.18) | |
Source | PubChem | |
URL | https://pubchem.ncbi.nlm.nih.gov | |
Description | Data deposited in or computed by PubChem | |
Molecular Formula |
C45H74O11 |
Source
|
Details | Computed by PubChem 2.1 (PubChem release 2019.06.18) | |
Source | PubChem | |
URL | https://pubchem.ncbi.nlm.nih.gov | |
Description | Data deposited in or computed by PubChem | |
Molecular Weight |
791.1 g/mol |
Source
|
Details | Computed by PubChem 2.1 (PubChem release 2021.05.07) | |
Source | PubChem | |
URL | https://pubchem.ncbi.nlm.nih.gov | |
Description | Data deposited in or computed by PubChem | |
Disclaimer and Information on In-Vitro Research Products
Please be aware that all articles and product information presented on BenchChem are intended solely for informational purposes. The products available for purchase on BenchChem are specifically designed for in-vitro studies, which are conducted outside of living organisms. In-vitro studies, derived from the Latin term "in glass," involve experiments performed in controlled laboratory settings using cells or tissues. It is important to note that these products are not categorized as medicines or drugs, and they have not received approval from the FDA for the prevention, treatment, or cure of any medical condition, ailment, or disease. We must emphasize that any form of bodily introduction of these products into humans or animals is strictly prohibited by law. It is essential to adhere to these guidelines to ensure compliance with legal and ethical standards in research and experimentation.