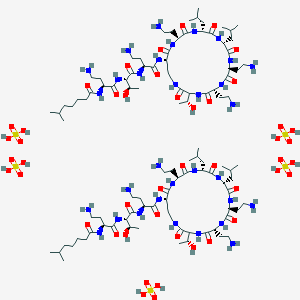
Colistin sulfate
Overview
Description
The compound “Colistin sulfate” is a complex chemical structure that includes multiple amino acids and sulfate groups. This compound is notable for its intricate arrangement of amino acids, which may have implications in various biochemical and industrial applications.
Preparation Methods
Synthetic Routes and Reaction Conditions
The synthesis of “Colistin sulfate” involves the stepwise assembly of amino acids through peptide bond formation. The process typically starts with the protection of amino groups to prevent unwanted reactions. The amino acids are then coupled using reagents such as carbodiimides or other coupling agents under controlled conditions. After the assembly of the peptide chain, the protecting groups are removed, and the compound is purified.
Industrial Production Methods
Industrial production of this compound may involve automated peptide synthesizers, which allow for the efficient and precise assembly of the peptide chain. The use of solid-phase peptide synthesis (SPPS) is common, where the peptide is assembled on a solid resin support. This method allows for easy purification and high yields.
Chemical Reactions Analysis
Types of Reactions
The compound “Colistin sulfate” can undergo various chemical reactions, including:
Oxidation: The amino acid residues can be oxidized under specific conditions.
Reduction: Reduction reactions can modify the disulfide bonds if present.
Substitution: Amino acid residues can be substituted with other functional groups.
Common Reagents and Conditions
Common reagents used in these reactions include oxidizing agents like hydrogen peroxide, reducing agents like dithiothreitol, and various coupling agents for substitution reactions. The conditions for these reactions vary but typically involve controlled temperatures and pH levels.
Major Products Formed
The major products formed from these reactions depend on the specific reagents and conditions used. For example, oxidation may lead to the formation of sulfoxides or sulfones, while reduction can result in the cleavage of disulfide bonds.
Scientific Research Applications
Chemistry
In chemistry, this compound can be used as a model peptide for studying peptide synthesis and reactions. Its complex structure makes it an excellent candidate for exploring new synthetic methods and reaction mechanisms.
Biology
In biology, the compound may be used to study protein-protein interactions, enzyme-substrate interactions, and other biochemical processes. Its unique arrangement of amino acids can provide insights into the structure-function relationships of peptides and proteins.
Medicine
In medicine, this compound may have potential applications in drug development and therapeutic research. Its ability to interact with specific molecular targets could make it a valuable tool for designing new drugs or understanding disease mechanisms.
Industry
In the industrial sector, this compound can be used in the development of new materials, such as biodegradable polymers or advanced biomaterials. Its unique properties may also find applications in biotechnology and nanotechnology.
Mechanism of Action
The mechanism of action of “Colistin sulfate” involves its interaction with specific molecular targets, such as enzymes or receptors. The compound’s amino acid residues can form hydrogen bonds, hydrophobic interactions, and electrostatic interactions with these targets, leading to various biological effects. The pathways involved may include signal transduction, metabolic regulation, and cellular communication.
Comparison with Similar Compounds
Similar Compounds
- “Unk-Dab-Thr-Dab-Dab(1)-Dab-D-Phe-Leu-Dab-Dab-Thr-(1).Unk-Dab-Thr-Dab-Dab(2)-Dab-D-Phe-Leu-Dab-Dab-Thr-(2).H2SO4”
- “Unk-Dab-Thr-Dab-Dab(1)-D-Dab-D-Phe-Leu-Dab-Dab-Thr-(1).Unk-Dab-Thr-Dab-Dab(2)-D-Dab-D-Phe-Leu-Dab-Dab-Thr-(2).H2SO4”
Uniqueness
The uniqueness of “Colistin sulfate” lies in its specific arrangement of amino acids and the presence of sulfate groups. This unique structure may confer distinct chemical and biological properties, making it valuable for various applications.
Biological Activity
Colistin sulfate, a polymyxin antibiotic, is primarily used to treat infections caused by multidrug-resistant Gram-negative bacteria. Its mechanism of action, pharmacokinetics, efficacy, and safety profile have been subjects of extensive research, particularly in the context of increasing antibiotic resistance.
This compound exerts its antibacterial effects by binding to the lipopolysaccharide (LPS) component of the bacterial outer membrane. This interaction disrupts the membrane integrity, leading to cell lysis and death. The drug is particularly effective against Gram-negative bacteria due to their unique cell wall structure.
Pharmacokinetics
This compound is poorly absorbed after oral administration, which limits its systemic bioavailability. Instead, it is often administered parenterally for serious infections. The pharmacokinetic profile indicates that this compound has a volume of distribution that varies based on the patient's condition and the presence of renal impairment.
Efficacy and Clinical Findings
Recent studies have highlighted the clinical efficacy of this compound in treating infections caused by carbapenem-resistant organisms (CROs). A retrospective study demonstrated a total effective rate of 74.6% in treating CRO infections, with a bacterial clearance rate of 72.6% . Notably, nephrotoxicity was observed in only 7.6% of cases, indicating a relatively favorable safety profile compared to other antibiotics used for similar infections .
Table 1: Summary of Clinical Efficacy Data for this compound
Study Reference | Total Effective Rate | Bacterial Clearance Rate | Nephrotoxicity Rate | Neurotoxicity Rate | 30-Day Mortality Rate |
---|---|---|---|---|---|
74.6% | 72.6% | 7.6% | 2.5% | 22.9% |
Combination Therapy
Research has indicated that combining this compound with other antimicrobial agents can enhance its effectiveness against resistant pathogens. For instance, a study explored the use of quinazoline compounds alongside colistin to potentiate its activity against Salmonella infections by targeting resistance mechanisms . This combination therapy reduced the minimum inhibitory concentration (MIC) of colistin significantly, demonstrating its potential as a therapeutic strategy.
Case Studies
- Case Study on Hematological Patients : In a cohort study involving hematological patients with CRO infections, this compound showed high clinical efficacy and low nephrotoxic risk when used in combination with other antimicrobials. This underscores the importance of tailored treatment regimens based on patient-specific factors .
- In Vivo Model : An in vivo model demonstrated that co-administration of colistin with CA439, a quinazoline compound, reduced Salmonella virulence significantly and enhanced bacterial clearance rates . This finding suggests that strategic combinations can improve treatment outcomes for resistant infections.
Safety Profile
While this compound is generally well-tolerated, monitoring for nephrotoxicity and neurotoxicity remains essential, particularly in patients with pre-existing renal conditions or those receiving high doses. Regular liver function tests and blood counts are recommended during treatment to mitigate risks associated with potential adverse effects .
Properties
IUPAC Name |
N-[(2S)-4-amino-1-[[(2S,3R)-1-[[(2S)-4-amino-1-oxo-1-[[(6R,9S,12R,15R,18S,21S)-6,9,18-tris(2-aminoethyl)-3-[(1R)-1-hydroxyethyl]-12,15-bis(2-methylpropyl)-2,5,8,11,14,17,20-heptaoxo-1,4,7,10,13,16,19-heptazacyclotricos-21-yl]amino]butan-2-yl]amino]-3-hydroxy-1-oxobutan-2-yl]amino]-1-oxobutan-2-yl]-6-methylheptanamide;sulfuric acid | |
---|---|---|
Details | Computed by LexiChem 2.6.6 (PubChem release 2019.06.18) | |
Source | PubChem | |
URL | https://pubchem.ncbi.nlm.nih.gov | |
Description | Data deposited in or computed by PubChem | |
InChI |
InChI=1S/2C52H98N16O13.5H2O4S/c2*1-27(2)11-9-10-12-40(71)59-32(13-19-53)47(76)68-42(31(8)70)52(81)64-35(16-22-56)44(73)63-37-18-24-58-51(80)41(30(7)69)67-48(77)36(17-23-57)61-43(72)33(14-20-54)62-49(78)38(25-28(3)4)66-50(79)39(26-29(5)6)65-45(74)34(15-21-55)60-46(37)75;5*1-5(2,3)4/h2*27-39,41-42,69-70H,9-26,53-57H2,1-8H3,(H,58,80)(H,59,71)(H,60,75)(H,61,72)(H,62,78)(H,63,73)(H,64,81)(H,65,74)(H,66,79)(H,67,77)(H,68,76);5*(H2,1,2,3,4)/t2*30-,31-,32+,33+,34+,35+,36-,37+,38-,39-,41?,42+;;;;;/m11...../s1 | |
Details | Computed by InChI 1.0.5 (PubChem release 2019.06.18) | |
Source | PubChem | |
URL | https://pubchem.ncbi.nlm.nih.gov | |
Description | Data deposited in or computed by PubChem | |
InChI Key |
ARWLUFSLVSQRNF-OTWIQOBPSA-N | |
Details | Computed by InChI 1.0.5 (PubChem release 2019.06.18) | |
Source | PubChem | |
URL | https://pubchem.ncbi.nlm.nih.gov | |
Description | Data deposited in or computed by PubChem | |
Canonical SMILES |
CC(C)CCCCC(=O)NC(CCN)C(=O)NC(C(C)O)C(=O)NC(CCN)C(=O)NC1CCNC(=O)C(NC(=O)C(NC(=O)C(NC(=O)C(NC(=O)C(NC(=O)C(NC1=O)CCN)CC(C)C)CC(C)C)CCN)CCN)C(C)O.CC(C)CCCCC(=O)NC(CCN)C(=O)NC(C(C)O)C(=O)NC(CCN)C(=O)NC1CCNC(=O)C(NC(=O)C(NC(=O)C(NC(=O)C(NC(=O)C(NC(=O)C(NC1=O)CCN)CC(C)C)CC(C)C)CCN)CCN)C(C)O.OS(=O)(=O)O.OS(=O)(=O)O.OS(=O)(=O)O.OS(=O)(=O)O.OS(=O)(=O)O | |
Details | Computed by OEChem 2.1.5 (PubChem release 2019.06.18) | |
Source | PubChem | |
URL | https://pubchem.ncbi.nlm.nih.gov | |
Description | Data deposited in or computed by PubChem | |
Isomeric SMILES |
C[C@H](C1C(=O)NCC[C@@H](C(=O)N[C@H](C(=O)N[C@@H](C(=O)N[C@@H](C(=O)N[C@H](C(=O)N[C@@H](C(=O)N1)CCN)CCN)CC(C)C)CC(C)C)CCN)NC(=O)[C@H](CCN)NC(=O)[C@H]([C@@H](C)O)NC(=O)[C@H](CCN)NC(=O)CCCCC(C)C)O.C[C@H](C1C(=O)NCC[C@@H](C(=O)N[C@H](C(=O)N[C@@H](C(=O)N[C@@H](C(=O)N[C@H](C(=O)N[C@@H](C(=O)N1)CCN)CCN)CC(C)C)CC(C)C)CCN)NC(=O)[C@H](CCN)NC(=O)[C@H]([C@@H](C)O)NC(=O)[C@H](CCN)NC(=O)CCCCC(C)C)O.OS(=O)(=O)O.OS(=O)(=O)O.OS(=O)(=O)O.OS(=O)(=O)O.OS(=O)(=O)O | |
Details | Computed by OEChem 2.1.5 (PubChem release 2019.06.18) | |
Source | PubChem | |
URL | https://pubchem.ncbi.nlm.nih.gov | |
Description | Data deposited in or computed by PubChem | |
Molecular Formula |
C104H206N32O46S5 | |
Details | Computed by PubChem 2.1 (PubChem release 2019.06.18) | |
Source | PubChem | |
URL | https://pubchem.ncbi.nlm.nih.gov | |
Description | Data deposited in or computed by PubChem | |
Molecular Weight |
2801.3 g/mol | |
Details | Computed by PubChem 2.1 (PubChem release 2021.05.07) | |
Source | PubChem | |
URL | https://pubchem.ncbi.nlm.nih.gov | |
Description | Data deposited in or computed by PubChem | |
Disclaimer and Information on In-Vitro Research Products
Please be aware that all articles and product information presented on BenchChem are intended solely for informational purposes. The products available for purchase on BenchChem are specifically designed for in-vitro studies, which are conducted outside of living organisms. In-vitro studies, derived from the Latin term "in glass," involve experiments performed in controlled laboratory settings using cells or tissues. It is important to note that these products are not categorized as medicines or drugs, and they have not received approval from the FDA for the prevention, treatment, or cure of any medical condition, ailment, or disease. We must emphasize that any form of bodily introduction of these products into humans or animals is strictly prohibited by law. It is essential to adhere to these guidelines to ensure compliance with legal and ethical standards in research and experimentation.