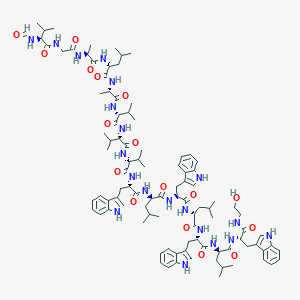
Gramicidin A
Overview
Description
Gramicidin A is a group of peptide antibiotics that includes this compound, B, C, D, and S. These compounds are primarily derived from the soil bacterium Brevibacillus brevis. This compound A, B, and C are linear peptides, while gramicidin S is a cyclic peptide. This compound is known for its ability to form ion channels in biological membranes, making it a potent antibiotic against gram-positive bacteria .
Mechanism of Action
Target of Action
Gramicidin A primarily targets bacterial membranes, with a strong preference for gram-positive cell membranes . It binds to and inserts itself into these membranes . The antimicrobial peptide can also target the Lipopolysaccharide layer of the cell membrane directly .
Mode of Action
This compound interacts with its targets by forming a transmembrane channel for monovalent cations (H+, Na+, and K+) . This channel disrupts the ion concentration gradients of the plasma membrane . It forces itself through the cell membrane, causing the contents of the cell to leak out and the surrounding contents to leak in through nano-sized tunnels called ion channels .
Biochemical Pathways
The disruption of ion concentration gradients by this compound affects various biochemical pathways. The diminished H+ gradient in the mitochondria inhibits ATP synthesis . This action is associated with the formation of hydroxyl radicals, possibly an end product of the transient depletion of NADH from the tricarboxylic acid cycle .
Pharmacokinetics
This compound is highly hemolytic, which prevents it from being administered internally . It is used primarily on the skin as a lotion or ointment, and in the treatment of infected surface wounds, and in eye, nose, and throat infections . The compound accumulates in mitochondria .
Result of Action
The action of this compound leads to several molecular and cellular effects. The resultant mitochondrial malfunction leads to mitophagy, while the cellular energy depletion induced G1 phase accumulation . These multiple events occur in a time-dependent fashion and ultimately cause potent inhibition of cell growth .
Biochemical Analysis
Biochemical Properties
Gramicidin A forms a β6.3-helix in a lipid bilayer and functions as a transmembrane channel for monovalent cations (H+, Na+, and K+) . It interacts with the membranes/lipid bilayers . The potent anticancer activity of this compound was believed to be mainly attributed to the free ion diffusion across the plasma membrane .
Cellular Effects
This compound not only disrupts the ion concentration gradients of the plasma membrane but also localizes in the mitochondria and depolarizes the inner mitochondrial membrane . The diminished H+ gradient in the mitochondria inhibits ATP synthesis . The resultant mitochondrial malfunction leads to mitophagy, while the cellular energy depletion induces G1 phase accumulation . The multiple events occur in a time-dependent fashion and ultimately cause potent inhibition of cell growth .
Molecular Mechanism
The molecular mechanism of this compound involves its sequence of alternating D- and L-chirality, which allows it to fold into a β6.3-helix in a lipid bilayer and form a head-to-head dimer . This structure functions as a transmembrane channel for monovalent cations (H+, Na+, and K+) . It disrupts ion concentration gradients, leading to various effects at the molecular level .
Temporal Effects in Laboratory Settings
In laboratory settings, the effects of this compound occur in a time-dependent fashion . It disrupts the ion concentration gradients of the plasma membrane, localizes in the mitochondria, depolarizes the inner mitochondrial membrane, inhibits ATP synthesis, leads to mitophagy, induces G1 phase accumulation, and ultimately inhibits cell growth .
Metabolic Pathways
This compound disrupts the H+ gradient in the mitochondria, which inhibits ATP synthesis . This suggests that it may be involved in the metabolic pathways related to ATP production.
Transport and Distribution
This compound is transported and distributed within cells and tissues by disrupting the ion concentration gradients of the plasma membrane . It also localizes in the mitochondria .
Subcellular Localization
The subcellular localization of this compound is primarily in the mitochondria . It localizes in the mitochondria and depolarizes the inner mitochondrial membrane .
Preparation Methods
Synthetic Routes and Reaction Conditions: Gramicidin A can be synthesized through nonribosomal peptide synthesis (NRPS), a process that does not involve ribosomes or messenger RNA. This method allows for the incorporation of non-standard amino acids and the formation of cyclic peptides .
Industrial Production Methods: Industrial production of gramicidin typically involves fermentation using Brevibacillus brevis. The fermentation broth is then subjected to extraction and purification processes. One efficient method involves using acidic ethanol as a solvent, which can extract up to 96% of gramicidin with a purity of 90% .
Chemical Reactions Analysis
Types of Reactions: Gramicidin A undergoes various chemical reactions, including:
Oxidation: this compound can be oxidized to form disulfide bonds, which can affect its structure and function.
Reduction: Reduction reactions can break disulfide bonds, altering the peptide’s conformation.
Substitution: Amino acid residues in gramicidin can be substituted to create analogs with different properties.
Common Reagents and Conditions:
Oxidizing Agents: Hydrogen peroxide, iodine.
Reducing Agents: Dithiothreitol (DTT), β-mercaptoethanol.
Substitution Reagents: Amino acid derivatives, coupling agents like EDC (1-ethyl-3-(3-dimethylaminopropyl)carbodiimide).
Major Products:
Oxidized this compound: Formation of disulfide bonds.
Reduced this compound: Linear peptides with free thiol groups.
Substituted this compound: Analog peptides with modified amino acid sequences.
Scientific Research Applications
Gramicidin A has a wide range of scientific research applications:
Chemistry: Used as a model system for studying ion channels and membrane permeability.
Biology: Investigated for its role in disrupting cellular ionic homeostasis.
Medicine: Applied in topical formulations for treating infected wounds and eye infections. .
Industry: Utilized in the development of biosensors and antimicrobial coatings.
Comparison with Similar Compounds
Gramicidin A can be compared with other antimicrobial peptides such as:
Polymyxin B: Another peptide antibiotic that targets gram-negative bacteria by disrupting their outer membrane.
Valinomycin: A cyclic peptide that forms potassium ion channels, similar to gramicidin’s ion channel formation but with a different ion selectivity.
Nisin: A lantibiotic that disrupts bacterial cell walls by binding to lipid II
Uniqueness of this compound: this compound’s ability to form ion channels in membranes and its specific action against gram-positive bacteria make it unique among antimicrobial peptides. Its linear and cyclic forms also provide versatility in its applications .
Biological Activity
Gramicidin A (gA) is a well-studied antimicrobial peptide renowned for its potent activity against Gram-positive bacteria. Its mechanism of action primarily involves the disruption of bacterial cell membranes, leading to cell lysis. This article delves into the biological activity of this compound, highlighting its mechanisms, efficacy against various pathogens, and recent research findings.
This compound operates through several key mechanisms:
- Membrane Permeabilization : this compound forms ion channels within bacterial membranes, disrupting ion gradients essential for cellular function. This is achieved by inserting itself into the lipid bilayer, creating pores that allow ions and small molecules to pass freely, ultimately leading to cell death .
- Radical Formation : Recent studies indicate that gA induces the formation of hydroxyl radicals, which contribute to oxidative stress within bacterial cells. This process is linked to the depletion of NADH from the tricarboxylic acid cycle, further exacerbating cellular damage and facilitating bacterial killing .
- Conformational Flexibility : The flexibility of gA plays a significant role in its ability to permeate membranes. Variations in peptide structure can influence its ion transport capabilities and overall antimicrobial effectiveness .
Antimicrobial Efficacy
This compound exhibits broad-spectrum antibacterial activity, particularly against Gram-positive bacteria such as Staphylococcus aureus, Streptococcus pneumoniae, and Enterococcus faecalis. The minimum inhibitory concentrations (MIC) for these bacteria are as follows:
Bacteria | MIC (nM) |
---|---|
Streptococcus pyogenes | 33 |
Enterococcus faecalis | 270 |
Streptococcus pneumoniae | 8.3 |
Streptococcus agalactiae | 1100 |
Listeria monocytogenes | 4300 |
These values demonstrate gA's potency against certain strains while highlighting variability in effectiveness across different species .
Case Studies and Research Findings
Several studies have explored the biological activity of this compound, revealing insights into its mechanisms and potential applications:
- Study on Staphylococcus aureus : Research utilizing atomic force microscopy and Fourier transform infrared spectroscopy outlined the morphological changes in S. aureus during gA treatment. The destruction process was categorized into four stages: pore formation, increased water permeability, bacterial flattening, and eventual lysis. This study emphasized gA's role in altering membrane composition by damaging lipids, proteins, and carbohydrates .
- Ion Channel Formation : Investigations into the structural properties of gA have shown that it forms a single channel within membranes rather than aggregating like other antimicrobial peptides. This unique mechanism may explain its high efficacy despite lower permeabilization rates compared to other peptides .
- Impact of Calcium Ions : Recent findings suggest that calcium ions can inhibit gA's antimicrobial activity at lower concentrations by blocking cation translocation across membranes. At higher concentrations, however, gA acts more like a detergent, leading to extensive membrane disruption .
Future Directions
Research continues to explore modifications of this compound to enhance its selectivity and reduce cytotoxicity towards mammalian cells. Recent studies have synthesized analogues with altered biological activity profiles while maintaining essential properties such as ion channel formation. These efforts aim to develop new therapeutic agents that leverage gA's antimicrobial properties while minimizing adverse effects .
Properties
IUPAC Name |
2-[2-[[2-[(2-formamido-3-methylbutanoyl)amino]acetyl]amino]propanoylamino]-N-[1-[[1-[[1-[[1-[[1-[[1-[[1-[[1-[[1-[[1-[[1-(2-hydroxyethylamino)-3-(1H-indol-3-yl)-1-oxopropan-2-yl]amino]-4-methyl-1-oxopentan-2-yl]amino]-3-(1H-indol-3-yl)-1-oxopropan-2-yl]amino]-4-methyl-1-oxopentan-2-yl]amino]-3-(1H-indol-3-yl)-1-oxopropan-2-yl]amino]-4-methyl-1-oxopentan-2-yl]amino]-3-(1H-indol-3-yl)-1-oxopropan-2-yl]amino]-3-methyl-1-oxobutan-2-yl]amino]-3-methyl-1-oxobutan-2-yl]amino]-3-methyl-1-oxobutan-2-yl]amino]-1-oxopropan-2-yl]-4-methylpentanamide | |
---|---|---|
Details | Computed by Lexichem TK 2.7.0 (PubChem release 2021.05.07) | |
Source | PubChem | |
URL | https://pubchem.ncbi.nlm.nih.gov | |
Description | Data deposited in or computed by PubChem | |
InChI |
InChI=1S/C99H140N20O17/c1-51(2)37-73(109-86(123)59(17)107-81(122)49-105-96(133)82(55(9)10)106-50-121)89(126)108-60(18)87(124)117-84(57(13)14)98(135)119-85(58(15)16)99(136)118-83(56(11)12)97(134)116-80(44-64-48-104-72-34-26-22-30-68(64)72)95(132)112-76(40-54(7)8)92(129)115-79(43-63-47-103-71-33-25-21-29-67(63)71)94(131)111-75(39-53(5)6)91(128)114-78(42-62-46-102-70-32-24-20-28-66(62)70)93(130)110-74(38-52(3)4)90(127)113-77(88(125)100-35-36-120)41-61-45-101-69-31-23-19-27-65(61)69/h19-34,45-48,50-60,73-80,82-85,101-104,120H,35-44,49H2,1-18H3,(H,100,125)(H,105,133)(H,106,121)(H,107,122)(H,108,126)(H,109,123)(H,110,130)(H,111,131)(H,112,132)(H,113,127)(H,114,128)(H,115,129)(H,116,134)(H,117,124)(H,118,136)(H,119,135) | |
Details | Computed by InChI 1.0.6 (PubChem release 2021.05.07) | |
Source | PubChem | |
URL | https://pubchem.ncbi.nlm.nih.gov | |
Description | Data deposited in or computed by PubChem | |
InChI Key |
ZWCXYZRRTRDGQE-UHFFFAOYSA-N | |
Details | Computed by InChI 1.0.6 (PubChem release 2021.05.07) | |
Source | PubChem | |
URL | https://pubchem.ncbi.nlm.nih.gov | |
Description | Data deposited in or computed by PubChem | |
Canonical SMILES |
CC(C)CC(C(=O)NC(C)C(=O)NC(C(C)C)C(=O)NC(C(C)C)C(=O)NC(C(C)C)C(=O)NC(CC1=CNC2=CC=CC=C21)C(=O)NC(CC(C)C)C(=O)NC(CC3=CNC4=CC=CC=C43)C(=O)NC(CC(C)C)C(=O)NC(CC5=CNC6=CC=CC=C65)C(=O)NC(CC(C)C)C(=O)NC(CC7=CNC8=CC=CC=C87)C(=O)NCCO)NC(=O)C(C)NC(=O)CNC(=O)C(C(C)C)NC=O | |
Details | Computed by OEChem 2.3.0 (PubChem release 2021.05.07) | |
Source | PubChem | |
URL | https://pubchem.ncbi.nlm.nih.gov | |
Description | Data deposited in or computed by PubChem | |
Molecular Formula |
C99H140N20O17 | |
Details | Computed by PubChem 2.1 (PubChem release 2021.05.07) | |
Source | PubChem | |
URL | https://pubchem.ncbi.nlm.nih.gov | |
Description | Data deposited in or computed by PubChem | |
Molecular Weight |
1882.3 g/mol | |
Details | Computed by PubChem 2.1 (PubChem release 2021.05.07) | |
Source | PubChem | |
URL | https://pubchem.ncbi.nlm.nih.gov | |
Description | Data deposited in or computed by PubChem | |
CAS No. |
11029-61-1, 1405-97-6 | |
Record name | Gramicidin A | |
Source | European Chemicals Agency (ECHA) | |
URL | https://echa.europa.eu/substance-information/-/substanceinfo/100.031.133 | |
Description | The European Chemicals Agency (ECHA) is an agency of the European Union which is the driving force among regulatory authorities in implementing the EU's groundbreaking chemicals legislation for the benefit of human health and the environment as well as for innovation and competitiveness. | |
Explanation | Use of the information, documents and data from the ECHA website is subject to the terms and conditions of this Legal Notice, and subject to other binding limitations provided for under applicable law, the information, documents and data made available on the ECHA website may be reproduced, distributed and/or used, totally or in part, for non-commercial purposes provided that ECHA is acknowledged as the source: "Source: European Chemicals Agency, http://echa.europa.eu/". Such acknowledgement must be included in each copy of the material. ECHA permits and encourages organisations and individuals to create links to the ECHA website under the following cumulative conditions: Links can only be made to webpages that provide a link to the Legal Notice page. | |
Record name | Gramicidin | |
Source | European Chemicals Agency (ECHA) | |
URL | https://echa.europa.eu/substance-information/-/substanceinfo/100.014.355 | |
Description | The European Chemicals Agency (ECHA) is an agency of the European Union which is the driving force among regulatory authorities in implementing the EU's groundbreaking chemicals legislation for the benefit of human health and the environment as well as for innovation and competitiveness. | |
Explanation | Use of the information, documents and data from the ECHA website is subject to the terms and conditions of this Legal Notice, and subject to other binding limitations provided for under applicable law, the information, documents and data made available on the ECHA website may be reproduced, distributed and/or used, totally or in part, for non-commercial purposes provided that ECHA is acknowledged as the source: "Source: European Chemicals Agency, http://echa.europa.eu/". Such acknowledgement must be included in each copy of the material. ECHA permits and encourages organisations and individuals to create links to the ECHA website under the following cumulative conditions: Links can only be made to webpages that provide a link to the Legal Notice page. | |
Q1: How does gramicidin exert its antibacterial activity?
A1: Gramicidin acts as an ionophore, specifically a channel former. It inserts into cell membranes, forming transmembrane channels that allow the passage of monovalent cations like potassium (K+) and sodium (Na+). [, , ] This disruption of ionic balance leads to cell dysfunction and death. [, , ] It primarily targets Gram-positive bacteria. [, , ]
Q2: Does the cyclic structure of gramicidin S impact its activity?
A2: Yes, research suggests the cyclic structure of gramicidin S is crucial for its immediate bacteriostatic effect. Acyclic decapeptide analogs of gramicidin S exhibited delayed bacteriostasis, suggesting a different mode of action compared to the cyclic parent compound. []
Q3: How does gramicidin affect mitochondrial function?
A3: Gramicidin D, a specific form of gramicidin, can induce respiration-independent swelling in corn mitochondria, potentially by acting on specific sites involved in substrate respiration. []
Q4: Can gramicidin affect mammalian cells?
A4: Yes, gramicidin can interact with mammalian cell membranes, impacting ion transport and potentially causing cell death. For instance, gramicidin A has shown to induce potassium leakage and sodium influx in human erythrocytes. [, ]
Q5: What is the structure of this compound?
A5: this compound is a linear pentadecapeptide with alternating L- and D-amino acids. In lipid bilayers, it forms a dimeric channel, likely a right-handed β6.3-helix, allowing the passage of ions. [, , ]
Q6: Does the conformation of this compound change upon ion binding?
A6: Yes, studies on this compound indicate that the indole rings of its tryptophan residues (Trp-11 and Trp-13) shift along the channel axis upon binding sodium ions (Na+), although their orientation remains relatively unchanged. []
Q7: Can gramicidin form complexes with metal ions?
A8: Yes, gramicidin can form complexes with various metal ions. For instance, studies revealed that two molecules of gramicidin S can bind to a calmodulin molecule in a calcium-dependent manner. [] Additionally, this compound can bind to divalent cations like manganese (Mn2+), primarily at the channel mouths, albeit blocking the passage of the divalent cations through the channel. []
Q8: How does the lipid environment affect gramicidin channel activity?
A10: The lipid composition of the membrane can significantly influence gramicidin channel activity. For example, gramicidin-mediated K+ flux is augmented in vesicles comprised of plasmenylcholine compared to phosphatidylcholine. This difference highlights the role of specific phospholipid subclasses in modulating channel function. []
Q9: Can gramicidin be incorporated into artificial systems for study?
A11: Yes, gramicidin is frequently incorporated into artificial lipid bilayers to study its channel-forming properties and ion transport mechanisms. These model systems allow for controlled manipulation of experimental conditions. [, , ]
Q10: Can gramicidin be immobilized for applications like drug screening?
A12: Yes, gramicidin can be immobilized within sol-gel-derived silica matrices while retaining its ion channel activity. This immobilization strategy holds potential for developing drug-screening and -sensing platforms. []
Q11: How do modifications at the N- and C-termini of gramicidin affect its activity?
A13: Modifications at the N- and C-termini can significantly alter gramicidin's channel-forming properties. For instance, introducing negative charges near the channel mouth, such as in O-pyromellitylgramicidin, enhances cation transport. Conversely, N-pyromellityldesformylgramicidin, with a modified N-terminus, loses its channel-forming ability. These findings support the head-to-head dimer model of the gramicidin channel. [, ]
Q12: What is the role of tryptophan residues in gramicidin function?
A14: Tryptophan residues are essential for gramicidin's channel formation and conductance. Replacing even a single tryptophan with another hydrophobic amino acid can significantly diminish channel activity. This highlights the importance of specific amino acid residues in maintaining the structure and function of gramicidin channels. [, , ]
Q13: Can the incorporation of unnatural amino acids impact gramicidin activity?
A15: Yes, incorporating unnatural amino acids can modify gramicidin's properties. For example, substituting D-phenylalanine with dehydrophenylalanine in gramicidin S resulted in an analog with enhanced β-sheet conformation and strong antimicrobial activity against Gram-positive bacteria. This demonstrates the potential for using unnatural amino acids to design gramicidin analogs with improved therapeutic profiles. []
Q14: Does gramicidin's effect on ion transport relate to its antimalarial activity?
A17: Studies suggest that gramicidin's ability to induce potassium leakage from infected erythrocytes may contribute to its antimalarial activity. The observation that gramicidin and its analog, tryptophan-N-formylated gramicidin (NFG), cause potassium efflux from Plasmodium falciparum-infected erythrocytes supports this hypothesis. []
Q15: What is the anticancer potential of gramicidin?
A18: Research indicates that gramicidin has potential as an anticancer agent. This compound exhibited potent antiproliferative effects on ovarian cancer cells, inducing apoptosis and inhibiting tumor growth in vitro and in vivo. These findings suggest that this compound could be a promising candidate for ovarian cancer therapy. [] Similar effects were observed in renal cell carcinoma (RCC), where this compound showed promising anticancer activity by inhibiting both oxidative phosphorylation and glycolysis, leading to energy depletion and cell death. []
Q16: What analytical techniques are used to study gramicidin?
A19: A variety of analytical techniques are employed to study gramicidin's structure, function, and interactions. These include, but are not limited to, circular dichroism (CD) spectroscopy, fluorescence spectroscopy, nuclear magnetic resonance (NMR) spectroscopy, X-ray crystallography, and various electrophysiological techniques. [, , , , , , , ]
Q17: How is the interaction between gramicidin and other molecules studied?
A20: Techniques like circular dichroism (CD) spectroscopy are valuable for studying the interaction of gramicidin with other molecules. For example, CD was used to study the interaction of gramicidin with the insecticide fenvalerate, revealing a stronger interaction with the channel-forming single-stranded β6.3-helix than with the double-helical form. []
Disclaimer and Information on In-Vitro Research Products
Please be aware that all articles and product information presented on BenchChem are intended solely for informational purposes. The products available for purchase on BenchChem are specifically designed for in-vitro studies, which are conducted outside of living organisms. In-vitro studies, derived from the Latin term "in glass," involve experiments performed in controlled laboratory settings using cells or tissues. It is important to note that these products are not categorized as medicines or drugs, and they have not received approval from the FDA for the prevention, treatment, or cure of any medical condition, ailment, or disease. We must emphasize that any form of bodily introduction of these products into humans or animals is strictly prohibited by law. It is essential to adhere to these guidelines to ensure compliance with legal and ethical standards in research and experimentation.