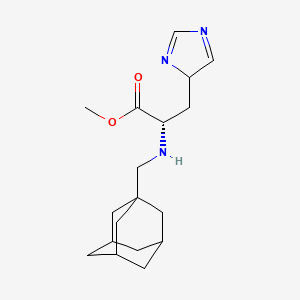
M2 ion channel blocker
Overview
Description
M2 ion channel blockers are compounds that inhibit the function of the M2 protein, an ion channel found in the influenza A virus. This protein is crucial for the virus’s replication process, as it facilitates proton conductance through the viral envelope and deacidification of the Golgi apparatus . By blocking this channel, M2 ion channel blockers prevent the virus from replicating, making them essential in antiviral therapies .
Preparation Methods
Synthetic Routes and Reaction Conditions: The synthesis of M2 ion channel blockers involves the creation of molecules that can effectively bind to the M2 transmembrane proton channel. One approach is the development of diazabicyclooctane derivatives with a constant positive charge of +2, which blocks proton diffusion through the channel . Molecular dynamics simulations are used to study the temperature fluctuations in the M2 structure and the ionization states of histidine residues at physiological pH values .
Industrial Production Methods: Industrial production of M2 ion channel blockers typically involves large-scale chemical synthesis processes. These processes are optimized for yield and purity, ensuring that the final product is effective in inhibiting the M2 ion channel. The production methods may include multiple steps of chemical reactions, purification, and quality control to meet pharmaceutical standards .
Chemical Reactions Analysis
Types of Reactions: M2 ion channel blockers primarily undergo binding reactions with the M2 protein. These reactions involve the formation of hydrogen bonds with key amino acid residues in the M2 channel, such as serine and histidine . The blockers create both steric and electrostatic barriers to proton transport through the channel .
Common Reagents and Conditions: The synthesis of M2 ion channel blockers often involves reagents that can introduce positive charges to the molecule, such as diazabicyclooctane derivatives . The reactions are typically carried out under controlled temperature and pH conditions to ensure the stability and effectiveness of the final product .
Major Products: The major products of these reactions are the M2 ion channel blockers themselves, which are designed to have high binding affinity for the M2 channel and effectively inhibit proton transport .
Scientific Research Applications
M2 ion channel blockers have a wide range of scientific research applications, particularly in the fields of chemistry, biology, medicine, and industry. In chemistry, they are used to study the mechanisms of ion channel inhibition and the design of new antiviral drugs . In biology, they help researchers understand the role of ion channels in cellular processes and viral replication . In medicine, M2 ion channel blockers are used as antiviral agents to treat influenza A infections . They are also explored for their potential use in other viral infections and diseases involving ion channel dysfunction .
Mechanism of Action
M2 ion channel blockers are compared with other antiviral drugs, such as amantadine and rimantadine . While amantadine and rimantadine also target the M2 ion channel, they have weaker effects on influenza A virus replication and are less effective against resistant strains . The new class of M2 ion channel blockers, such as diazabicyclooctane derivatives, offers advantages in terms of binding affinity and effectiveness . These new blockers have a positive charge of +2, which creates a positive electrostatic potential barrier to proton transport, making them more effective than traditional M2 ion channel blockers .
Comparison with Similar Compounds
- Amantadine
- Rimantadine
- Diazabicyclooctane derivatives
By understanding the unique properties and applications of M2 ion channel blockers, researchers can continue to develop more effective antiviral therapies and explore new avenues for treating viral infections.
Properties
IUPAC Name |
methyl (2S)-2-(1-adamantylmethylamino)-3-(4H-imidazol-4-yl)propanoate | |
---|---|---|
Details | Computed by Lexichem TK 2.7.0 (PubChem release 2021.05.07) | |
Source | PubChem | |
URL | https://pubchem.ncbi.nlm.nih.gov | |
Description | Data deposited in or computed by PubChem | |
InChI |
InChI=1S/C18H27N3O2/c1-23-17(22)16(5-15-9-19-11-21-15)20-10-18-6-12-2-13(7-18)4-14(3-12)8-18/h9,11-16,20H,2-8,10H2,1H3/t12?,13?,14?,15?,16-,18?/m0/s1 | |
Details | Computed by InChI 1.0.6 (PubChem release 2021.05.07) | |
Source | PubChem | |
URL | https://pubchem.ncbi.nlm.nih.gov | |
Description | Data deposited in or computed by PubChem | |
InChI Key |
KKMJGUDIUVOQTI-STWSTGMMSA-N | |
Details | Computed by InChI 1.0.6 (PubChem release 2021.05.07) | |
Source | PubChem | |
URL | https://pubchem.ncbi.nlm.nih.gov | |
Description | Data deposited in or computed by PubChem | |
Canonical SMILES |
COC(=O)C(CC1C=NC=N1)NCC23CC4CC(C2)CC(C4)C3 | |
Details | Computed by OEChem 2.3.0 (PubChem release 2021.05.07) | |
Source | PubChem | |
URL | https://pubchem.ncbi.nlm.nih.gov | |
Description | Data deposited in or computed by PubChem | |
Isomeric SMILES |
COC(=O)[C@H](CC1C=NC=N1)NCC23CC4CC(C2)CC(C4)C3 | |
Details | Computed by OEChem 2.3.0 (PubChem release 2021.05.07) | |
Source | PubChem | |
URL | https://pubchem.ncbi.nlm.nih.gov | |
Description | Data deposited in or computed by PubChem | |
Molecular Formula |
C18H27N3O2 | |
Details | Computed by PubChem 2.1 (PubChem release 2021.05.07) | |
Source | PubChem | |
URL | https://pubchem.ncbi.nlm.nih.gov | |
Description | Data deposited in or computed by PubChem | |
Molecular Weight |
317.4 g/mol | |
Details | Computed by PubChem 2.1 (PubChem release 2021.05.07) | |
Source | PubChem | |
URL | https://pubchem.ncbi.nlm.nih.gov | |
Description | Data deposited in or computed by PubChem | |
Retrosynthesis Analysis
AI-Powered Synthesis Planning: Our tool employs the Template_relevance Pistachio, Template_relevance Bkms_metabolic, Template_relevance Pistachio_ringbreaker, Template_relevance Reaxys, Template_relevance Reaxys_biocatalysis model, leveraging a vast database of chemical reactions to predict feasible synthetic routes.
One-Step Synthesis Focus: Specifically designed for one-step synthesis, it provides concise and direct routes for your target compounds, streamlining the synthesis process.
Accurate Predictions: Utilizing the extensive PISTACHIO, BKMS_METABOLIC, PISTACHIO_RINGBREAKER, REAXYS, REAXYS_BIOCATALYSIS database, our tool offers high-accuracy predictions, reflecting the latest in chemical research and data.
Strategy Settings
Precursor scoring | Relevance Heuristic |
---|---|
Min. plausibility | 0.01 |
Model | Template_relevance |
Template Set | Pistachio/Bkms_metabolic/Pistachio_ringbreaker/Reaxys/Reaxys_biocatalysis |
Top-N result to add to graph | 6 |
Feasible Synthetic Routes
Disclaimer and Information on In-Vitro Research Products
Please be aware that all articles and product information presented on BenchChem are intended solely for informational purposes. The products available for purchase on BenchChem are specifically designed for in-vitro studies, which are conducted outside of living organisms. In-vitro studies, derived from the Latin term "in glass," involve experiments performed in controlled laboratory settings using cells or tissues. It is important to note that these products are not categorized as medicines or drugs, and they have not received approval from the FDA for the prevention, treatment, or cure of any medical condition, ailment, or disease. We must emphasize that any form of bodily introduction of these products into humans or animals is strictly prohibited by law. It is essential to adhere to these guidelines to ensure compliance with legal and ethical standards in research and experimentation.