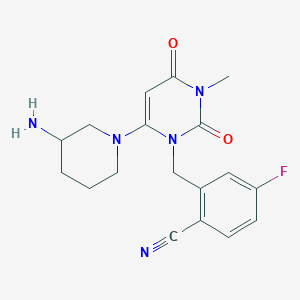
TRELAGLIPTIN
Overview
Description
Trelagliptin (brand name Zafatek®) is a long-acting, once-weekly dipeptidyl peptidase-4 (DPP-4) inhibitor approved in Japan for the treatment of type 2 diabetes mellitus (T2DM). It selectively inhibits DPP-4, an enzyme responsible for degrading incretin hormones like glucagon-like peptide-1 (GLP-1) and glucose-dependent insulinotropic polypeptide (GIP). By prolonging incretin activity, this compound enhances glucose-dependent insulin secretion and suppresses glucagon release, thereby improving glycemic control [1]. Its unique pharmacokinetic profile allows weekly dosing, which improves patient adherence compared to daily DPP-4 inhibitors like sitagliptin or alogliptin [19].
Preparation Methods
Chlorination-Condensation-Ammonolysis Route
The foundational synthetic route for trelagliptin, as disclosed in patent CN105541793A, involves three sequential steps: chlorination, condensation, and ammonolysis .
Step 1: Chlorination of 2-Hydroxymethyl-4-Fluorobenzonitrile
The process begins with the conversion of 2-hydroxymethyl-4-fluorobenzonitrile to 2-chloromethyl-4-fluorobenzonitrile using thionyl chloride (SOCl₂) in toluene at 60°C. This step achieves near-quantitative yield (98–100%) while avoiding hazardous reagents like phosphorus pentachloride . The chloro intermediate exhibits superior stability compared to bromo analogs, reducing decomposition risks during storage.
Step 2: Condensation with 6-Chloro-3-Methyluracil
In a pivotal condensation reaction, 2-chloromethyl-4-fluorobenzonitrile reacts with 6-chloro-3-methyluracil in N-methylpyrrolidone (NMP) using N,N-diisopropylethylamine (DIPEA) as a base. Optimized conditions (60°C for 1 hour) yield 82% of 2-[(6-chloro-3,4-dihydro-3-methyl-2,4-dioxo-1(2H)-pyrimidyl)methyl]-4-fluorobenzonitrile with 97.8% purity . Key parameters include:
-
Molar ratio : 1:1 for chloro intermediate and uracil derivative
-
Solvent system : NMP enables efficient mixing and heat transfer
-
Workup : Crystallization from isopropyl alcohol removes unreacted starting materials
Step 3: Ammonolysis to this compound Free Base
The final ammonolysis employs aqueous ammonia in tetrahydrofuran (THF) at 25°C, replacing traditional sodium methoxide-mediated reactions. This modification reduces byproduct formation, yielding this compound free base with 95% purity after recrystallization .
Nucleophilic Substitution Approach with Boc-Protected Intermediates
Patent CN104961726A introduces a Boc (tert-butyloxycarbonyl)-protected pathway to minimize amine-related side reactions .
Alkylation of 6-Chloro-3-Methyluracil
2-Cyano-5-fluorobenzyl bromide reacts with 6-chloro-3-methyluracil in dimethylformamide (DMF) using potassium carbonate as base. At 70°C, this step achieves 98.9% yield with 99.7% purity, significantly reducing dimeric impurities compared to earlier methods .
Nucleophilic Displacement with (R)-3-Boc-Aminopiperidine
The key substitution reaction employs (R)-3-Boc-aminopiperidine in dichloromethane with triethylamine. Phase-transfer catalysis (tetrabutylammonium bromide) enhances reaction rate, completing displacement in 4 hours at 40°C . Boc deprotection using trifluoroacetic acid (TFA) in THF yields this compound free base (85.5% yield, 99.6% purity) .
Comparative Data: Protection Strategies
Parameter | Boc Protection | Direct Amination |
---|---|---|
Yield | 85.5% | 82% |
Purity | 99.6% | 97.8% |
Byproducts | <0.5% | 2.2% |
Reaction Time | 4 hours | 1 hour |
Bromination and Alkylation Optimization
The ACS Publications route optimizes bromination of 4-fluoro-2-methylbenzonitrile using N-bromosuccinimide (NBS) and azobisisobutyronitrile (AIBN) in carbon tetrachloride . Key advancements include:
-
Radical initiator : AIBN (1 mol%) enables complete conversion at 80°C
-
Solvent system : CCl₄ minimizes polybromination byproducts
-
Workup : Sequential washing with sodium thiosulfate and sodium bicarbonate removes excess bromine
The resultant 2-bromomethyl-4-fluorobenzonitrile undergoes alkylation with 6-chloro-3-methyluracil in acetonitrile using potassium carbonate, achieving 94% yield . This method circumvents the need for expensive transition metal catalysts.
Mechanochemical Salinization for this compound Succinate
Recent advances in green chemistry demonstrate solvent-free synthesis of this compound succinate via ball milling :
Direct Milling of this compound Solvates
Milling this compound ethanolate (TRE-Et) with succinic acid (1:1 molar ratio) for 45 minutes at 25 Hz produces this compound succinate (TRE-S) with 99.2% purity. The mechanism involves:
-
Solvate dissociation during mechanical grinding
-
Proton transfer from succinic acid to this compound’s piperidine nitrogen
-
Co-crystallization via hydrogen bonding networks
Comparative Efficiency of Salinization Methods
Method | Time | Purity | Solvent Used |
---|---|---|---|
Solution Crystallization | 8 hours | 98.5% | Ethanol |
Mechanochemical | 45 min | 99.2% | None |
Liquid-Assisted Grinding | 2 hours | 98.8% | Methanol |
Impurity Profiling and Control Strategies
Major Process-Related Impurities
-
Dimeric Byproducts : Formed during condensation steps due to over-alkylation. Controlled by maintaining stoichiometric ratios and low temperatures .
-
Enantiomeric Contaminants : Generated during piperidine coupling. Chiral HPLC analysis (Lux Cellulose-2 column) ensures enantiomeric excess >99.9% .
-
Degradation Products : Acidic conditions produce this compound Acid Degradation Product (TAD), mitigated through pH-controlled workups .
Analytical Quality Control
-
HPLC Methods :
-
Mass Spectrometry : ESI-MS confirms intermediate structures (e.g., m/z 294.04 for condensation product)
Step | Traditional Solvent | Green Alternative |
---|---|---|
Chlorination | Toluene | Cyclopentyl methyl ether |
Condensation | NMP | 2-MeTHF |
Crystallization | Isopropyl alcohol | Ethanol/water |
Waste Reduction Strategies
-
Catalyst Recycling : DIPEA recovery via acid-base extraction (85% recovery rate)
-
Mother Liquor Reuse : Up to 3 cycles in crystallization steps without yield loss
-
Emissions Control : Scrubbers for HCl and NH₃ vapors during ammonolysis
Industrial Scale-Up Challenges
Critical Process Parameters
-
Temperature Control : ±2°C tolerance in exothermic condensation steps
-
Mixing Efficiency : Reynolds number >10,000 for slurry reactions
-
Drying Protocols : Fluidized bed drying at 45°C prevents polymorphic transitions
Cost Analysis
Component | Cost Contribution |
---|---|
Starting Materials | 58% |
Solvent Recovery | 22% |
Energy Consumption | 15% |
Waste Treatment | 5% |
Chemical Reactions Analysis
Types of Reactions
2-[6-(3-Amino-piperidin-1-yl)-3-methyl-2,4-dioxo-3,4-dihydro-2H-pyrimidin-1-ylmethyl]-4-fluoro-benzonitrile undergoes various types of chemical reactions, including:
Oxidation: The compound can be oxidized to form corresponding oxides or other oxidation products.
Reduction: Reduction reactions can lead to the formation of reduced derivatives.
Substitution: The compound can undergo nucleophilic or electrophilic substitution reactions, particularly at the piperidine and pyrimidine rings.
Cyclization: Intramolecular cyclization reactions can lead to the formation of additional ring structures.
Common Reagents and Conditions
Common reagents used in these reactions include oxidizing agents (e.g., hydrogen peroxide, potassium permanganate), reducing agents (e.g., sodium borohydride, lithium aluminum hydride), and various nucleophiles and electrophiles. Reaction conditions such as temperature, solvent, and pH are optimized to achieve the desired products.
Major Products
The major products formed from these reactions depend on the specific reaction conditions and reagents used. For example, oxidation reactions may yield oxides, while substitution reactions can lead to the formation of substituted derivatives with different functional groups.
Scientific Research Applications
Glycemic Control
Numerous studies have demonstrated the efficacy of trelagliptin in lowering hemoglobin A1c (HbA1c) levels. For instance, a phase 3 study showed that patients switching from daily DPP-4 inhibitors to this compound maintained stable glycemic control without significant adverse effects. In this study, HbA1c decreased significantly from baseline, indicating effective glucose management .
Table 1: Summary of Clinical Trials Evaluating this compound
Study Type | Population | Duration | Dosage | HbA1c Reduction (%) | Adverse Events |
---|---|---|---|---|---|
Phase II | 55 patients | 12 weeks | 100 mg/week | -0.71 (vs placebo) | 72.7% mild to moderate |
Phase III | 200 patients | 52 weeks | 100 mg/week | -0.72 (vs placebo) | 61.5% mild to moderate |
Open-label | Diabetic patients switched from daily DPP-4 inhibitors | 12 weeks | 100 mg/week | Stable control | Mild/moderate events only |
Safety Profile
This compound has been shown to have a favorable safety profile compared to other antidiabetic medications. In clinical trials, the incidence of treatment-emergent adverse events (TEAEs) was primarily mild to moderate. Serious adverse events were rare, and no cases of severe hypoglycemia were reported . This safety profile makes this compound an attractive option for patients who may be sensitive to side effects from other diabetes medications.
Patient Compliance and Quality of Life
The once-weekly dosing regimen of this compound is designed to improve patient adherence to therapy. Studies indicate that reducing the frequency of medication can lead to better compliance and improved overall quality of life for patients with T2DM . The convenience of a weekly dose helps mitigate the treatment burden often associated with diabetes management.
Case Studies
Case Study 1: Transition from Daily DPP-4 Inhibitor
A clinical trial involving patients previously stabilized on daily sitagliptin therapy showed that switching to this compound maintained glycemic control without significant fluctuations in blood glucose levels. Patients reported high satisfaction with the once-weekly regimen, highlighting improved adherence and quality of life .
Case Study 2: Long-term Efficacy
In a long-term study over 52 weeks, this compound was administered as monotherapy or in combination with other oral antidiabetic drugs. Results indicated sustained reductions in HbA1c levels and consistent safety outcomes throughout the study period .
Mechanism of Action
The mechanism of action of 2-[6-(3-Amino-piperidin-1-yl)-3-methyl-2,4-dioxo-3,4-dihydro-2H-pyrimidin-1-ylmethyl]-4-fluoro-benzonitrile involves its interaction with specific molecular targets and pathways. The compound may act as an inhibitor or activator of certain enzymes, receptors, or signaling pathways. For example, it may inhibit dipeptidyl peptidase-4 (DPP-4) activity, leading to increased levels of glucagon-like peptide-1 (GLP-1) and improved glucose tolerance . The exact molecular targets and pathways depend on the specific biological context and the compound’s structure-activity relationship.
Comparison with Similar Compounds
Comparison with Similar DPP-4 Inhibitors
Pharmacokinetic and Pharmacodynamic Properties
Trelagliptin exhibits distinct advantages in potency and duration of action compared to other DPP-4 inhibitors:
- Potency : this compound is 4–12× more potent than alogliptin and sitagliptin in vitro, achieving sustained DPP-4 inhibition (~70% at 168 hours post-dose) with lower plasma concentrations (1.43 ng/mL for 50% inhibition vs. 6.6 ng/mL for alogliptin) [18].
- Binding Mechanism: Unlike covalent inhibitors (e.g., saxagliptin), this compound binds reversibly and competitively to DPP-4 via non-covalent interactions, contributing to its slower dissociation rate [11].
Efficacy in Glycemic Control
- HbA1c Reduction: In T2DM patients, this compound 100 mg/week demonstrated non-inferiority to alogliptin 25 mg/day, reducing HbA1c by 0.72% over 12 weeks in renal-impaired patients and 0.84% in combination with insulin therapy [16].
- Sustained Action : this compound maintains DPP-4 inhibition for 7–9 days post-dose, comparable to omarigliptin but superior to daily agents [10].
Special Populations
- Renal Impairment : this compound’s clearance decreases by 65–79% in severe renal impairment (vs. 35–50% for sitagliptin), but its 25 mg/week dose maintains efficacy without increasing hypoglycemia risk [3].
- Hepatic Impairment: No dose adjustment required due to non-hepatic metabolism [22].
Biological Activity
Trelagliptin (SYR-472, marketed as Zafatek) is a novel dipeptidyl peptidase-4 (DPP-4) inhibitor primarily developed for the treatment of type 2 diabetes mellitus. This article delves into the biological activity of this compound, focusing on its pharmacological mechanisms, effects on various biological pathways, and implications for clinical applications.
This compound exhibits potent inhibition of DPP-4, an enzyme that degrades incretin hormones. By inhibiting DPP-4, this compound enhances the levels of incretins such as GLP-1 (glucagon-like peptide-1), which in turn stimulates insulin secretion and suppresses glucagon release. This dual action contributes to improved glycemic control in diabetic patients.
Inhibition Potency
Research indicates that this compound is approximately 4-fold more potent than alogliptin and 12-fold more potent than sitagliptin in inhibiting human DPP-4. It also shows over 10,000-fold selectivity for DPP-4 compared to related proteases such as DPP-8 and DPP-9 . The inhibition mechanism is characterized as reversible and competitive, with a dissociation half-life of about 30 minutes .
Osteoblastic Differentiation
Recent studies have shown that this compound promotes osteoblastic differentiation through the activation of the AMPK signaling pathway. In vitro experiments using MC3T3-E1 cells demonstrated that this compound significantly increased alkaline phosphatase (ALP) activity and calcium deposition, markers indicative of osteoblast differentiation .
Key Findings:
- ALP Activity Increase : Treatment with this compound resulted in a significant elevation in ALP activity.
- Calcified Nodules Formation : Enhanced formation of calcified nodules was observed in treated cells.
- Gene Expression : Upregulation of osteoblastic markers such as ALP, OCN (osteocalcin), OPN (osteopontin), and BMP-2 was noted upon treatment with this compound.
The activation of AMPK was confirmed as crucial for these effects, as inhibition of AMPK with compound C negated the beneficial outcomes induced by this compound .
Pharmacokinetics and Bioavailability
This compound's pharmacokinetic profile supports its once-weekly dosing regimen. Clinical trials have shown sustained inhibition of DPP-4 activity lasting up to 7 days post-administration, which is advantageous for patient compliance .
Comparative Bioavailability Data
Formulation Type | Bioavailability (%) |
---|---|
This compound Suspension Film | 175% relative to commercial tablets |
Commercial Tablets | 39.37% |
This enhanced bioavailability is attributed to its formulation as a fast-dissolving oral drug, improving absorption and therapeutic efficacy .
Clinical Implications and Case Studies
This compound has been evaluated in various clinical settings, demonstrating significant improvements in glycemic control without notable adverse effects. Its unique pharmacological profile allows it to be a viable option for patients who may benefit from less frequent dosing schedules.
Case Study Overview
- Patient Cohort : Type 2 diabetes patients inadequately controlled on other therapies.
- Outcome Measures : HbA1c levels, fasting plasma glucose levels.
- Results : Significant reductions in HbA1c levels were observed after 24 weeks of treatment with this compound.
Q & A
Basic Research Questions
Q. What experimental methods are used to characterize Trelagliptin’s mechanism of action as a DPP-4 inhibitor?
this compound’s inhibition mechanism is studied using kinetic analysis to determine its binding kinetics (e.g., dissociation half-life ≈30 minutes) and X-ray crystallography to confirm non-covalent interactions with DPP-4 . Competitive inhibition is validated via Lineweaver-Burk plots under varying substrate concentrations, demonstrating direct competition with the substrate for the DPP-4 active site . Selectivity profiling against related proteases (e.g., DPP-8/9) ensures specificity, with this compound showing >10,000-fold selectivity for DPP-4 .
Q. How do researchers design clinical trials to compare this compound’s efficacy with daily DPP-4 inhibitors?
Trials employ randomized, double-blind, active-controlled designs with non-inferiority endpoints. For example, in a Phase 3 study, 240 subjects were randomized into this compound and daily DPP-4 inhibitor groups, using HbA1c and quality-of-life (DTR-QOL) scores as primary endpoints . Efficacy is analyzed via ANCOVA models adjusted for baseline covariates (e.g., HbA1c stratification), ensuring statistical rigor .
Q. What in vitro assays are used to evaluate this compound’s metabolic stability?
Metabolic stability is assessed using rat or human liver microsomes incubated with this compound (1 µM) in phosphate buffer (pH 7.4) containing NADPH. LC/MS analysis quantifies parent compound degradation over time (0–30 minutes), with results normalized to control incubations .
Advanced Research Questions
Q. How is sample size determined for this compound trials to ensure statistical power?
Sample size calculations assume effect sizes (e.g., 19.0% vs. 14.4% improvement in DTR-QOL scores), standard deviation (SD=12.1%) , and 80% power at a 5% significance level. A discontinuation rate (e.g., 8%) is factored in, requiring ≥110 subjects per group (total N=240 after adjustment) .
Q. How can researchers resolve contradictions in reported DPP-4 inhibition potency across studies?
Discrepancies are addressed by standardizing kinetic assay conditions (e.g., pre-incubation time, substrate concentrations) and validating findings with structural data (e.g., X-ray crystallography to confirm binding interactions) . Comparative studies using shared reference inhibitors (e.g., alogliptin, sitagliptin) ensure consistency in IC₅₀ measurements .
Q. What methodological considerations are critical for analyzing this compound’s non-covalent binding to DPP-4?
X-ray diffraction at high resolution (≤2.0 Å) is essential to visualize binding interactions without covalent modifications. Surface plasmon resonance (SPR) or isothermal titration calorimetry (ITC) can quantify binding affinity (KD), while molecular dynamics simulations model conformational stability .
Q. How do researchers address variability in patient-reported outcomes (e.g., quality of life) in this compound trials?
Factor analysis of multi-item questionnaires (e.g., DTR-QOL) isolates domains (e.g., symptom burden, treatment satisfaction). Mixed-effects models account for intra-patient variability, and sensitivity analyses test robustness against missing data .
Properties
IUPAC Name |
2-[[6-(3-aminopiperidin-1-yl)-3-methyl-2,4-dioxopyrimidin-1-yl]methyl]-4-fluorobenzonitrile | |
---|---|---|
Details | Computed by LexiChem 2.6.6 (PubChem release 2019.06.18) | |
Source | PubChem | |
URL | https://pubchem.ncbi.nlm.nih.gov | |
Description | Data deposited in or computed by PubChem | |
InChI |
InChI=1S/C18H20FN5O2/c1-22-17(25)8-16(23-6-2-3-15(21)11-23)24(18(22)26)10-13-7-14(19)5-4-12(13)9-20/h4-5,7-8,15H,2-3,6,10-11,21H2,1H3 | |
Details | Computed by InChI 1.0.5 (PubChem release 2019.06.18) | |
Source | PubChem | |
URL | https://pubchem.ncbi.nlm.nih.gov | |
Description | Data deposited in or computed by PubChem | |
InChI Key |
IWYJYHUNXVAVAA-UHFFFAOYSA-N | |
Details | Computed by InChI 1.0.5 (PubChem release 2019.06.18) | |
Source | PubChem | |
URL | https://pubchem.ncbi.nlm.nih.gov | |
Description | Data deposited in or computed by PubChem | |
Canonical SMILES |
CN1C(=O)C=C(N(C1=O)CC2=C(C=CC(=C2)F)C#N)N3CCCC(C3)N | |
Details | Computed by OEChem 2.1.5 (PubChem release 2019.06.18) | |
Source | PubChem | |
URL | https://pubchem.ncbi.nlm.nih.gov | |
Description | Data deposited in or computed by PubChem | |
Molecular Formula |
C18H20FN5O2 | |
Details | Computed by PubChem 2.1 (PubChem release 2019.06.18) | |
Source | PubChem | |
URL | https://pubchem.ncbi.nlm.nih.gov | |
Description | Data deposited in or computed by PubChem | |
Molecular Weight |
357.4 g/mol | |
Details | Computed by PubChem 2.1 (PubChem release 2021.05.07) | |
Source | PubChem | |
URL | https://pubchem.ncbi.nlm.nih.gov | |
Description | Data deposited in or computed by PubChem | |
Synthesis routes and methods
Procedure details
Disclaimer and Information on In-Vitro Research Products
Please be aware that all articles and product information presented on BenchChem are intended solely for informational purposes. The products available for purchase on BenchChem are specifically designed for in-vitro studies, which are conducted outside of living organisms. In-vitro studies, derived from the Latin term "in glass," involve experiments performed in controlled laboratory settings using cells or tissues. It is important to note that these products are not categorized as medicines or drugs, and they have not received approval from the FDA for the prevention, treatment, or cure of any medical condition, ailment, or disease. We must emphasize that any form of bodily introduction of these products into humans or animals is strictly prohibited by law. It is essential to adhere to these guidelines to ensure compliance with legal and ethical standards in research and experimentation.