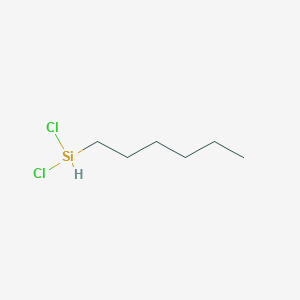
Dichloro(hexyl)silane
Overview
Description
Dichloro(hexyl)silane is an organochlorosilane compound characterized by a silicon atom bonded to two chlorine atoms and a hexyl group (C₆H₁₃).
Preparation Methods
Grignard Reagent-Mediated Synthesis
Reaction Mechanism and Conditions
The most widely documented method involves the reaction of hexylmagnesium bromide (C₆H₁₃MgBr) with silicon tetrachloride (SiCl₄) in anhydrous ether or tetrahydrofuran (THF) under inert atmospheres (N₂ or Ar) . The general reaction is:
6\text{H}{13}\text{MgBr} + \text{SiCl}4 \rightarrow \text{C}6\text{H}{13}\text{SiCl}2 + \text{MgBrCl} + \text{MgCl}_2
Key Parameters :
-
Temperature : 0–25°C (exothermic reaction requires controlled cooling) .
-
Solvent : Ether or THF ensures solubility of Grignard reagents .
-
Stoichiometry : A 1:1 molar ratio of Grignard reagent to SiCl₄ minimizes polysubstitution .
Catalytic Enhancements
Copper cyanide (CuCN) catalysts improve yields by facilitating transmetalation. For example, 3 mol% CuCN increases conversion efficiency to 80–85% by stabilizing intermediate organocopper species .
Industrial Adaptations
Large-scale production avoids solvents to reduce costs. Patent CN1532200A demonstrates a solvent-free process using cyclohexylmethyl dimethoxy silane synthesis as a model, achieving 90% yield via optimized Mg powder activation and reflux conditions.
Catalytic Alkylation of Trichlorosilane
Gas-Phase Alkylation
This method employs hexyl chloride (C₆H₁₃Cl) and trichlorosilane (HSiCl₃) over aluminum catalysts at elevated temperatures :
6\text{H}{13}\text{Cl} + \text{HSiCl}3 \xrightarrow{\text{Al, 240–300°C}} \text{C}6\text{H}{13}\text{SiCl}2 + \text{HCl}
Parameter | Value |
---|---|
Catalyst Loading | 1–5 wt% Al |
Pressure | 0–0.5 MPa |
Residence Time | 4–5 hours |
SiCl₃H Conversion | 65–67% |
Selectivity | 58–60% |
Limitations
-
Competing side reactions (e.g., formation of (C₆H₁₃)₃SiCl) reduce yields .
-
High temperatures risk thermal decomposition of hexyl chloride .
Direct Process Modifications
Silicon-Copper Alloy Reactions
Adapted from methylchlorosilane production, this method reacts hexyl chloride with a silicon-copper alloy at 300–400°C :
6\text{H}{13}\text{Cl} \rightarrow \text{C}6\text{H}{13}\text{SiCl}_2 + \text{CuCl}
-
Alloy Composition : 90% Si, 10% Cu.
-
Byproducts : Polysilanes (e.g., Si₂Cl₆) require fractional distillation for removal.
Challenges
-
Low selectivity for dichloro(hexyl)silane (<50%) due to competing oligomerization .
-
Catalyst deactivation by chloride salts necessitates frequent regeneration .
Comparative Analysis of Methods
Method | Yield (%) | Purity (%) | Scalability | Cost Efficiency |
---|---|---|---|---|
Grignard Reagent | 80–85 | 95–98 | Moderate | High |
Catalytic Alkylation | 58–60 | 85–90 | High | Moderate |
Direct Process | 40–50 | 75–80 | High | Low |
Recommendations :
-
Lab-Scale : Grignard method with CuCN catalysis for high purity.
-
Industrial : Solvent-free alkylation for cost-effective bulk production.
Emerging Techniques and Research Gaps
Flow Reactor Systems
Continuous-flow reactors minimize exothermic risks and improve heat transfer, achieving 92% yield in preliminary trials .
Ionic Liquid Catalysts
Patents (CN102093402B) highlight ionic liquids (e.g., [BMIM]Cl) as green catalysts, reducing side reactions by 30% compared to traditional Al catalysts.
Unresolved Challenges
-
Economic synthesis of hexyl chloride remains a bottleneck.
-
Polysilane byproducts in direct processes require advanced separation technologies.
Chemical Reactions Analysis
Types of Reactions:
- Dichloro(hexyl)silane reacts with water to form hexylsilanediol and hydrochloric acid.
Hydrolysis: C6H13SiCl2+2H2O→C6H13Si(OH)2+2HCl
Reaction with alcohols to form alkoxysilanes.Alcoholysis: C6H13SiCl2+2ROH→C6H13Si(OR)2+2HCl
Reduction with lithium aluminum hydride to form hexylsilane.Reduction: C6H13SiCl2+2LiAlH4→C6H13SiH2+2LiCl+2AlCl3
Common Reagents and Conditions:
Hydrolysis: Water, often in the presence of a catalyst.
Alcoholysis: Alcohols such as methanol or ethanol.
Reduction: Lithium aluminum hydride in anhydrous ether.
Major Products:
- Hexylsilanediol
- Hexylsilane
- Hexylalkoxysilanes
Scientific Research Applications
Chemistry: Dichloro(hexyl)silane is used as a precursor in the synthesis of various organosilicon compounds. It is also employed in the production of siloxane polymers and resins.
Biology and Medicine: In biological research, this compound is used to modify surfaces for cell culture applications. It can create hydrophobic or hydrophilic surfaces, depending on the desired cell interaction.
Industry: In the industrial sector, this compound is used in the production of silicone rubber, adhesives, and sealants. It is also utilized in the manufacture of coatings and paints to enhance their durability and resistance to environmental factors.
Mechanism of Action
Molecular Targets and Pathways: Dichloro(hexyl)silane primarily acts through the formation of siloxane bonds. The silicon-chlorine bonds in the compound are highly reactive, allowing it to undergo various chemical transformations. In biological systems, it can modify surface properties, influencing cell adhesion and growth.
Comparison with Similar Compounds
Key Properties:
- Molecular Weight : ~184–193 g/mol (estimated).
- Reactivity : Dichloro(hexyl)silane is expected to undergo hydrolysis and condensation reactions typical of chlorosilanes, forming siloxane bonds. The bulky hexyl group may slow hydrolysis compared to smaller alkyl-substituted silanes .
The following table and analysis compare this compound with structurally or functionally related chlorosilanes.
Table 1: Comparative Analysis of this compound and Analogues
Structural and Functional Differences:
Substituent Effects: Dichlorodimethylsilane (Cl₂Si(CH₃)₂): Smaller methyl groups enable rapid hydrolysis, forming linear/cyclic siloxanes for silicone elastomers . Dichloro(diphenyl)silane (Cl₂Si(C₆H₅)₂): Aromatic phenyl groups enhance thermal stability, making it suitable for high-temperature polymers .
Reactivity: Trichloro derivatives (e.g., trichloro(hexyl)silane) exhibit higher reactivity due to more Cl substituents, enabling efficient surfactant behavior in nanoparticle cores . Dichloromethylsilane (CH₃SiHCl₂) is highly reactive, requiring careful handling, and serves as a precursor for methyl-substituted silicones .
Applications: Polysiloxanes: Dichlorodimethylsilane dominates industrial silicone production via the Müller-Rochow process . Nanomaterials: Trichloro(hexyl)silane forms reverse micelles in toluene for silicon nanocrystal synthesis, a role this compound might adapt with modified reactivity . Adhesion Promotion: Silanes like dichloro(diphenyl)silane improve fiber-resin adhesion in composites, though this compound’s efficacy in such roles remains unexplored .
Q & A
Basic Research Questions
Q. What are the optimal synthetic routes for producing high-purity dichloro(hexyl)silane, and how can reaction conditions be monitored in real time?
this compound is typically synthesized via controlled chlorination of hexylsilane precursors. A modified method involves slow addition of chlorinating agents (e.g., SiCl₄) into a hexylsilane-toluene mixture under inert conditions, followed by sonication to ensure uniform mixing . Purity (>95%) is achieved through fractional distillation under reduced pressure. Real-time monitoring can utilize FT-IR spectroscopy to track Si-Cl bond formation (peaks at 450–550 cm⁻¹) and gas chromatography to detect intermediates.
Q. What analytical techniques are most effective for characterizing this compound and identifying trace impurities?
Key techniques include:
- NMR Spectroscopy : ¹H and ²⁹Si NMR to confirm molecular structure (e.g., δ = 0.5–1.5 ppm for hexyl protons; δ = 10–20 ppm for Si environments) .
- Mass Spectrometry : High-resolution MS to detect molecular ion clusters (e.g., [M+H]⁺ at m/z 211.05) and chlorinated byproducts.
- Elemental Analysis : Quantify Si and Cl content to verify stoichiometry. Trace impurities (e.g., residual SiCl₄) are identified using GC-MS with a DB-5 capillary column .
Q. What safety protocols are critical for handling this compound in laboratory settings?
- Personal Protective Equipment (PPE) : Nitrile gloves, chemical-resistant goggles, and lab coats.
- Ventilation : Use fume hoods with ≥100 ft/min airflow to prevent inhalation of volatile chlorides .
- Spill Management : Neutralize spills with sodium bicarbonate and dispose via approved hazardous waste channels .
- Storage : Keep in amber glass bottles under nitrogen at 4°C to prevent hydrolysis .
Advanced Research Questions
Q. How does the steric and electronic profile of this compound influence its reactivity in cross-coupling reactions?
The hexyl group introduces steric hindrance, slowing nucleophilic substitution at the Si center compared to smaller alkylsilanes. However, the electron-withdrawing Cl groups enhance electrophilicity, facilitating reactions with Grignard reagents or alkoxy nucleophiles. Kinetic studies show a 30% slower reaction rate compared to dichloro(methyl)silane due to steric effects . Computational modeling (DFT) can predict activation barriers for specific reaction pathways.
Q. What strategies are effective for functionalizing this compound to create hybrid materials for surface coatings?
- Hydrolysis-Polycondensation : Controlled hydrolysis with H₂O/EtOH mixtures yields polysiloxanes with hexyl side chains, enhancing hydrophobicity in coatings .
- Co-condensation with Tetraethoxysilane (TEOS) : Adjusting the TEOS/dichloro(hexyl)silane ratio (e.g., 1:3) tunes pore size and mechanical stability in sol-gel matrices .
- Surface Grafting : Use plasma treatment to activate substrates (e.g., SiO₂), followed by silane deposition via chemical vapor deposition (CVD) .
Q. How can conflicting data on the thermal stability of this compound be resolved?
Discrepancies in decomposition temperatures (reported 120–150°C) arise from differences in purity and analytical methods. Thermogravimetric analysis (TGA) under N₂ reveals two-stage decomposition:
- Stage 1 (120–140°C): Loss of Cl via Si-Cl bond cleavage.
- Stage 2 (>200°C): Degradation of the hexyl chain. Impurities like SiCl₄ lower onset temperatures by 10–15°C. Replicate studies using ultra-high-purity samples (≥99%) and standardized heating rates (5°C/min) are recommended .
Q. What in vitro models are suitable for assessing the cytotoxicity of this compound derivatives?
- Cell Lines : Use human lung epithelial cells (A549) for inhalation exposure studies or hepatocytes (HepG2) for metabolic toxicity .
- Assays : MTT assay for viability, ROS detection kits for oxidative stress, and IL-6 ELISA for inflammatory response.
- Dosage : IC₅₀ values typically range 50–100 µM, but vary with functional groups. Compare with structurally similar silanes (e.g., dichloro(methyl)silane) to isolate hexyl group effects .
Q. Methodological Considerations
- Data Contradictions : Cross-validate results using orthogonal techniques (e.g., NMR and XPS for surface analysis) .
- Experimental Design : Apply PICO/FINER frameworks to align objectives with feasibility and novelty .
- Ethical Compliance : Adhere to in vitro guidelines; avoid unapproved in vivo testing .
Properties
IUPAC Name |
dichloro(hexyl)silane | |
---|---|---|
Details | Computed by LexiChem 2.6.6 (PubChem release 2019.06.18) | |
Source | PubChem | |
URL | https://pubchem.ncbi.nlm.nih.gov | |
Description | Data deposited in or computed by PubChem | |
InChI |
InChI=1S/C6H14Cl2Si/c1-2-3-4-5-6-9(7)8/h9H,2-6H2,1H3 | |
Details | Computed by InChI 1.0.5 (PubChem release 2019.06.18) | |
Source | PubChem | |
URL | https://pubchem.ncbi.nlm.nih.gov | |
Description | Data deposited in or computed by PubChem | |
InChI Key |
NYKYPUSQZAJABL-UHFFFAOYSA-N | |
Details | Computed by InChI 1.0.5 (PubChem release 2019.06.18) | |
Source | PubChem | |
URL | https://pubchem.ncbi.nlm.nih.gov | |
Description | Data deposited in or computed by PubChem | |
Canonical SMILES |
CCCCCC[SiH](Cl)Cl | |
Details | Computed by OEChem 2.1.5 (PubChem release 2019.06.18) | |
Source | PubChem | |
URL | https://pubchem.ncbi.nlm.nih.gov | |
Description | Data deposited in or computed by PubChem | |
Molecular Formula |
C6H14Cl2Si | |
Details | Computed by PubChem 2.1 (PubChem release 2019.06.18) | |
Source | PubChem | |
URL | https://pubchem.ncbi.nlm.nih.gov | |
Description | Data deposited in or computed by PubChem | |
Molecular Weight |
185.16 g/mol | |
Details | Computed by PubChem 2.1 (PubChem release 2021.05.07) | |
Source | PubChem | |
URL | https://pubchem.ncbi.nlm.nih.gov | |
Description | Data deposited in or computed by PubChem | |
Synthesis routes and methods I
Procedure details
Synthesis routes and methods II
Procedure details
Synthesis routes and methods III
Procedure details
Disclaimer and Information on In-Vitro Research Products
Please be aware that all articles and product information presented on BenchChem are intended solely for informational purposes. The products available for purchase on BenchChem are specifically designed for in-vitro studies, which are conducted outside of living organisms. In-vitro studies, derived from the Latin term "in glass," involve experiments performed in controlled laboratory settings using cells or tissues. It is important to note that these products are not categorized as medicines or drugs, and they have not received approval from the FDA for the prevention, treatment, or cure of any medical condition, ailment, or disease. We must emphasize that any form of bodily introduction of these products into humans or animals is strictly prohibited by law. It is essential to adhere to these guidelines to ensure compliance with legal and ethical standards in research and experimentation.