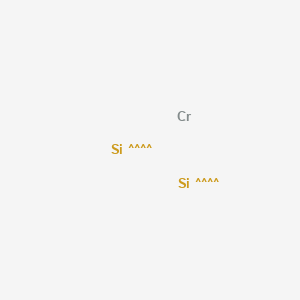
CHROMIUM SILICIDE
- Click on QUICK INQUIRY to receive a quote from our team of experts.
- With the quality product at a COMPETITIVE price, you can focus more on your research.
Overview
Description
CHROMIUM SILICIDE is a useful research compound. Its molecular formula is CrSi2 and its molecular weight is 108.17 g/mol. The purity is usually 95%.
BenchChem offers high-quality this compound suitable for many research applications. Different packaging options are available to accommodate customers' requirements. Please inquire for more information about this compound including the price, delivery time, and more detailed information at info@benchchem.com.
Mechanism of Action
Target of Action
Chromium Silicide (CrSi) primarily targets silicon (Si) atoms in the solid phase . The interaction between chromium and silicon is a key aspect of the compound’s action. Chromium in silicon has an increased reactivity , which is crucial for the formation of chromium silicides.
Mode of Action
The interaction between chromium and silicon leads to the formation of chromium silicides. This process involves the diffusion of silicon atoms in the solid phase . Chromium silicides are formed through currentless diffusion saturation, metal-thermal reduction, and electrochemical behaviors of chromium- and silicon-containing melts . The combined reduction of chromium (III) chloride and sodium fluorine-silicate by metallic sodium or magnesium makes it possible to obtain silicide powders .
Biochemical Pathways
Chromium silicides are formed through several pathways, including currentless diffusion saturation, metal-thermal reduction of the compounds of metals from groups IV–VIB and silicon with alkaline and alkaline-earth metals, and electrochemical synthesis (ES) from the melts . These processes lead to the formation of chromium silicides in the form of superfine powders and coatings .
Pharmacokinetics
The formation of chromium silicides involves the diffusion of silicon atoms in the solid phase, suggesting that the compound’s bioavailability may be influenced by this process .
Result of Action
The result of this compound’s action is the formation of chromium silicides in the form of superfine powders and coatings . These silicides are characterized by their valuable physicochemical properties and high thermal and chemical stability . They are also environmentally safe materials .
Action Environment
The action of this compound is influenced by environmental factors such as temperature and the presence of other compounds. For instance, the synthesis of chromium silicides requires high temperatures . Additionally, the presence of other compounds, such as chromium (III) chloride and sodium fluorine-silicate, influences the formation of chromium silicides .
Biological Activity
Chromium silicide (CrSi) is a compound of chromium and silicon that has garnered attention due to its potential applications in various fields, including electronics, thermoelectric materials, and nanotechnology. Understanding its biological activity is crucial for assessing its safety and efficacy in these applications. This article aims to explore the biological activity of this compound, supported by relevant research findings, data tables, and case studies.
This compound can be synthesized through various methods, including molecular beam epitaxy (MBE) and chemical vapor deposition (CVD). The formation of different phases such as CrSi2, CrSi, and Cr5Si3 during synthesis can influence its properties and biological interactions.
Table 1: Phases of this compound Synthesized
Sample ID | Substrate Temp. (°C) | Si Cell Temp. (°C) | Majority Phase | Minority Phases |
---|---|---|---|---|
1 | 900 | 1500 | CrSi2 | CrSi, Cr5Si3 |
2 | 1000 | 1500 | CrSi2 | CrSi, Cr5Si3 |
3 | 600 | 1525 | CrSi2 | CrSi |
Antimicrobial Properties
Research indicates that chromium compounds, particularly chromium(III) complexes, exhibit significant antimicrobial activity. Studies show that these complexes can effectively combat bacterial strains such as Staphylococcus aureus and Escherichia coli by inducing oxidative stress, which leads to bacterial cell damage . this compound's potential role in this context remains an area for further exploration.
The antimicrobial effects of chromium compounds are attributed to their ability to generate reactive oxygen species (ROS), which can damage bacterial DNA and cellular components. This oxidative damage is a critical factor in the bactericidal activity observed in chromium(III) complexes .
Case Study: this compound in Nanostructures
Recent studies have explored the fabrication of this compound nanostructures within silicon nanopillars. These nanostructures demonstrate enhanced field-emission behavior, suggesting potential applications in electronic devices . However, the biological implications of these nanostructures require careful examination to assess their safety in biological systems.
Toxicological Considerations
The biotoxicity of chromium compounds is well-documented, particularly concerning environmental exposure. Chromium(III) is generally considered less toxic than chromium(VI), but chronic exposure can still lead to adverse health effects . Understanding the toxicity profile of this compound is essential for evaluating its safety in biomedical applications.
Table 2: Toxicity Data for Chromium Compounds
Compound | Toxicity Level | Health Effects |
---|---|---|
Chromium(III) | Low | Skin irritation, allergic reactions |
Chromium(VI) | High | Carcinogenic effects, respiratory issues |
Future Research Directions
Given the promising properties of this compound, future research should focus on:
- In Vivo Studies : Investigating the biological interactions and toxicity of this compound in living organisms.
- Mechanistic Studies : Elucidating the mechanisms underlying its antimicrobial activity.
- Nanotechnology Applications : Exploring the implications of this compound nanostructures in biomedical devices.
Properties
CAS No. |
12018-09-6 |
---|---|
Molecular Formula |
CrSi2 |
Molecular Weight |
108.17 g/mol |
InChI |
InChI=1S/Cr.2Si |
InChI Key |
CHXGWONBPAADHP-UHFFFAOYSA-N |
SMILES |
[Si].[Si].[Cr] |
Canonical SMILES |
[Si]#[Cr]#[Si] |
Origin of Product |
United States |
Q1: How can chromium silicide be formed?
A1: this compound can be formed through various methods, including:
- Ion-beam mixing: This technique involves bombarding a chromium/silicon bilayer with argon ions, leading to the intermixing of the elements and subsequent silicide formation. []
- Thermal annealing: Heating a chromium film deposited on a silicon substrate promotes silicon diffusion into the chromium layer, resulting in silicide formation. [, , , ]
- Pack cementation: This process utilizes a powder pack containing chromium and silicon sources to deposit this compound onto a substrate at high temperatures. []
- Electrochemical synthesis: Chromium silicides can be synthesized electrochemically in molten salt systems containing chromium and silicon precursors. [, ]
- Mechanical alloying: This method uses mechanical energy to induce chemical reactions between chromium and silicon powders, ultimately forming this compound. []
Q2: Does the substrate material influence the formation of this compound?
A: Yes, the substrate material can influence the chemical composition and oxidation state of chromium within the silicide film. For instance, this compound films deposited on silicon substrates exhibit different oxide contents and chromium oxidation states compared to those deposited on glass or silicon nitride substrates. []
Q3: What happens to inert gases like krypton during this compound formation?
A: The behavior of implanted inert gases during silicide formation depends on their initial location. Krypton implanted in the chromium film remains stationary relative to the chromium during silicide growth. In contrast, krypton implanted in the silicon substrate accumulates at the interface between the silicon and the growing silicide. []
Q4: What are the common stoichiometries of this compound?
A: Several this compound phases exist, including Cr3Si, Cr5Si3, CrSi, and CrSi2. Each phase exhibits distinct structural and electronic properties, making them suitable for different applications. [, , , ]
Q5: How does the silicon content affect the properties of this compound?
A: Increasing the silicon content generally leads to changes in the material's electronic structure and bonding characteristics. For example, higher silicon content in chromium silicides correlates with: * Weaker autoionization contributions in the Cr-M2,3 VV Auger spectra []* Narrower Cr-M2,3 absorption structures that approach the edge energy []
Q6: What are the advantages of using this compound in high-temperature applications?
A: this compound exhibits high melting points and excellent oxidation resistance, making it suitable for high-temperature applications. This is particularly relevant in areas like thermoelectric energy conversion and high-temperature sensors. [, ]
Q7: How does the presence of this compound affect the performance of amorphous silicon solar cells?
A: Introducing thin layers of this compound at the interfaces between metal electrodes and doped regions in amorphous silicon solar cells can significantly enhance their performance. This improvement stems from the reduction in activation energy (~0.225 eV) observed in both n-doped and p-doped amorphous silicon films when in contact with this compound. This reduction in activation energy contributes to both built-in and open-circuit voltage enhancements. [, , ]
Q8: Can this compound enhance the stability of field emitter arrays?
A: Yes, applying a this compound coating to silicon field emitters improves their stability by reducing current fluctuations. This stabilization arises from the reduced number of chemically active sites on the silicide-protected surface, leading to a chemically stable layer with higher electrical conductivity. []
Q9: Does this compound exhibit catalytic activity?
A: While the provided research doesn't specifically focus on the catalytic properties of this compound, its presence influences chemical reactions. For instance, in the SiC-Cr-Si system, this compound formation can occur through the reduction of silica (SiO2) by silicon and silicon carbide, suggesting a potential catalytic role for this compound in this reaction. []
Q10: Have theoretical calculations been employed to study chromium silicides?
A10: Yes, first-principles calculations based on density functional theory have been used to predict various properties of chromium silicides, including:
- Chemical stability: Enthalpies and binding energies calculations provide insights into the thermodynamic stability of different this compound phases. []
- Mechanical properties: Calculations of elastic moduli, such as bulk modulus, shear modulus, and Young’s modulus, reveal the mechanical strength and stiffness of these compounds. []
- Electronic properties: Density of states calculations provide valuable information regarding the electronic band structure and bonding characteristics of chromium silicides. []
- Thermodynamic properties: The Debye temperature, related to the vibrations of atoms within the material, can be calculated using theoretical models like the Debye quasi-harmonic approximation. []
Q11: What are the environmental implications of using this compound?
A11: While the provided research doesn't directly address the environmental impact of this compound, it's crucial to consider the potential environmental implications of its production, use, and disposal. Assessing its ecotoxicological effects and developing strategies for responsible waste management and recycling are important for ensuring environmental sustainability.
Q12: Are there alternative materials to this compound for similar applications?
A: Yes, depending on the specific application, several alternative materials exist. For instance, other metal silicides, like tungsten silicide, exhibit comparable high-temperature properties and are explored for thermoelectric applications. [, ] Similarly, other materials, such as titanium nitride (TiN), are investigated as diffusion barriers in microelectronic applications. [] The choice of the most suitable material depends on factors like cost, performance requirements, and specific application needs.
Disclaimer and Information on In-Vitro Research Products
Please be aware that all articles and product information presented on BenchChem are intended solely for informational purposes. The products available for purchase on BenchChem are specifically designed for in-vitro studies, which are conducted outside of living organisms. In-vitro studies, derived from the Latin term "in glass," involve experiments performed in controlled laboratory settings using cells or tissues. It is important to note that these products are not categorized as medicines or drugs, and they have not received approval from the FDA for the prevention, treatment, or cure of any medical condition, ailment, or disease. We must emphasize that any form of bodily introduction of these products into humans or animals is strictly prohibited by law. It is essential to adhere to these guidelines to ensure compliance with legal and ethical standards in research and experimentation.