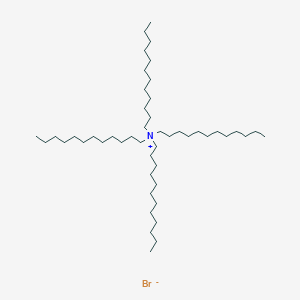
Tetradodecylammonium bromide
Overview
Description
Tetradodecylammonium bromide, also known as tetralaurylammonium bromide, is a quaternary ammonium salt. It consists of a positively charged nitrogen atom attached to four long alkyl chains, each containing twelve carbon atoms. This compound is commonly used as a surfactant and phase-transfer catalyst due to its ability to facilitate the transfer of reactants between aqueous and organic phases .
Mechanism of Action
Tetradodecylammonium bromide (TDAB) is a quaternary ammonium salt that plays a significant role in various biochemical processes . This article will delve into the mechanism of action of TDAB, covering its primary targets, mode of action, biochemical pathways, pharmacokinetics, results of action, and the influence of environmental factors on its action.
Target of Action
TDAB is a surfactant and phase-transfer catalyst . As a surfactant, it reduces surface tension between two liquids or a liquid and a solid, facilitating their interaction. As a phase-transfer catalyst, it enables the migration of a reactant from one phase into another where reaction occurs .
Mode of Action
The mode of action of TDAB is primarily based on its surfactant and phase-transfer catalyst properties . As a surfactant, it alters the properties of water molecules, reducing surface tension and enabling better interaction between different phases of a solution. As a phase-transfer catalyst, it facilitates the transfer of a reactant from one phase to another, thereby enhancing the efficiency of the reaction .
Biochemical Pathways
As a phase-transfer catalyst, it is known to play a role in various chemical reactions, including the synthesis of ethyl-lophine .
Pharmacokinetics
Given its use as a surfactant and phase-transfer catalyst, it is likely to have significant interactions with biological membranes, potentially influencing its absorption and distribution .
Result of Action
The primary result of TDAB’s action is the facilitation of chemical reactions through its role as a phase-transfer catalyst .
Action Environment
The action of TDAB can be influenced by various environmental factors. For instance, the efficiency of its action as a phase-transfer catalyst can be affected by the polarity of the solvent, temperature, and pressure
Biochemical Analysis
Biochemical Properties
Tetradodecylammonium bromide has been found to interact with mixed perovskite films in biochemical reactions . The XPS and FTIR analysis validated that this compound binds to the mixed perovskite through hydrogen bonding .
Cellular Effects
In the context of perovskite solar cells, this compound has been used as a post-surface modifier to suppress the density of defects from the mixed perovskite film . This modification has shown to enhance the performance of the cells .
Molecular Mechanism
The molecular mechanism of this compound involves its binding to the mixed perovskite through hydrogen bonding . This interaction forms a capping layer on the surface of the 3D perovskite .
Temporal Effects in Laboratory Settings
This compound has shown to form a hydrophobic capping surface and safeguards the underlayer perovskite from moisture . As a result, the modified perovskite solar cells have exhibited almost no performance loss after 30 days in air .
Preparation Methods
Synthetic Routes and Reaction Conditions: Tetradodecylammonium bromide can be synthesized through the quaternization of dodecylamine with dodecyl bromide. The reaction typically occurs in an organic solvent such as chloroform or toluene, under reflux conditions. The reaction is as follows:
C12H25NH2+4C12H25Br→[C12H25]4NBr+3HBr
Industrial Production Methods: In industrial settings, the production of this compound involves similar synthetic routes but on a larger scale. The process is optimized for higher yields and purity, often involving continuous flow reactors and advanced purification techniques such as recrystallization and chromatography .
Types of Reactions:
Substitution Reactions: this compound can undergo nucleophilic substitution reactions where the bromide ion is replaced by other nucleophiles.
Phase-Transfer Catalysis: It acts as a catalyst in phase-transfer reactions, facilitating the transfer of reactants between immiscible phases.
Common Reagents and Conditions:
Nucleophiles: Common nucleophiles include hydroxide ions, cyanide ions, and alkoxide ions.
Conditions: Reactions are typically carried out in biphasic systems with vigorous stirring to ensure efficient phase transfer.
Major Products:
- The major products depend on the nucleophile used. For example, using hydroxide ions would yield tetradodecylammonium hydroxide.
Chemistry:
Phase-Transfer Catalysis: Widely used in organic synthesis to enhance reaction rates and yields.
Surfactant: Employed in the formulation of detergents and emulsifiers.
Biology and Medicine:
Antimicrobial Agent: Exhibits antimicrobial properties, making it useful in disinfectants and antiseptics.
Industry:
Electroplating: Used in the electroplating industry to improve the quality of metal coatings.
Oil Recovery: Applied in enhanced oil recovery processes to improve the extraction of oil from reservoirs.
Comparison with Similar Compounds
Tetraoctadecylammonium Bromide: Similar structure but with longer alkyl chains (eighteen carbon atoms).
Tetraheptylammonium Bromide: Similar structure but with shorter alkyl chains (seven carbon atoms).
Tetrakis(decyl)ammonium Bromide: Similar structure but with ten carbon atoms in each alkyl chain.
Uniqueness: Tetradodecylammonium bromide is unique due to its balance between hydrophobic and hydrophilic properties, making it an effective surfactant and phase-transfer catalyst. Its twelve-carbon alkyl chains provide optimal solubility and micelle formation, which are crucial for its applications in various fields .
Biological Activity
Tetradodecylammonium bromide (TDAB) is a quaternary ammonium compound that has garnered attention for its diverse biological activities and applications in various fields, including analytical chemistry, biochemistry, and material science. This article explores the biological activity of TDAB, highlighting its mechanisms of action, potential therapeutic uses, and safety considerations.
Chemical Structure and Properties
TDAB is characterized by a long hydrophobic alkyl chain (C12) attached to a quaternary ammonium group. Its structure can be represented as:
This configuration imparts unique surfactant properties to TDAB, making it effective in various applications, including as an ionophore in selective electrodes and in taste sensor technology.
Mechanisms of Biological Activity
-
Ion Selectivity and Sensor Applications :
TDAB has been utilized in the development of ion-selective electrodes (ISEs), particularly for nitrate detection. A study demonstrated that TDAB serves as an effective ionophore in PVC membranes, enhancing the electrode's sensitivity and selectivity for nitrate ions. The optimal composition of the membrane was found to be 2:65:33 (ionophore:DOP:PVC), yielding a Nernstian slope of -54 ± 1.0 mV/decade, with a detection range from 10 µM to 1 mM . -
Taste Sensor Development :
In another innovative application, TDAB was incorporated into taste sensors to assess bitterness in pharmaceuticals. The sensors showed a robust correlation between predicted bitterness and actual sensory data, indicating that TDAB can effectively interact with bitter compounds to modulate their perceived taste . -
Thermodynamic Promoter in Clathrate Hydrates :
Recent studies have explored the role of TDAB as a thermodynamic promoter in the formation of semi-clathrate hydrates. By reducing the pressure-temperature requirements for gas hydrate formation, TDAB could facilitate more efficient gas storage and transportation methods .
Case Studies
- Nitrate Ion-Selective Electrode : The development of a novel nitrate ISE using TDAB demonstrated enhanced performance metrics compared to traditional electrodes. The study highlighted the importance of the membrane composition in achieving optimal sensitivity and selectivity for nitrate ions, which is crucial for environmental monitoring .
- Bitterness Assessment : A study assessing the bitterness intensity of various medications found that TDAB-based sensors could accurately predict bitterness levels, significantly impacting pharmaceutical development aimed at improving drug palatability .
Table: Comparison of Ion Selectivity in Sensors
Sensor Type | Ionophore Used | Selectivity Coefficient (F⁻) | Nernstian Slope (mV/decade) |
---|---|---|---|
TDAB Sensor | TDAB | -10.64 | -54 ± 1.0 |
TOMACl Sensor | TOMACl | -7.01 | - |
OAm Sensor | OAm | -0.61 | - |
Safety and Toxicology
Despite its beneficial applications, TDAB poses certain health risks upon exposure. Chronic exposure may lead to respiratory issues such as pneumoconiosis due to inhalation of fine dust particles . Additionally, animal studies have indicated potential developmental toxicity associated with high doses of bromides, including those similar to TDAB . It is crucial for researchers and practitioners to observe safety protocols when handling this compound.
Properties
IUPAC Name |
tetradodecylazanium;bromide | |
---|---|---|
Source | PubChem | |
URL | https://pubchem.ncbi.nlm.nih.gov | |
Description | Data deposited in or computed by PubChem | |
InChI |
InChI=1S/C48H100N.BrH/c1-5-9-13-17-21-25-29-33-37-41-45-49(46-42-38-34-30-26-22-18-14-10-6-2,47-43-39-35-31-27-23-19-15-11-7-3)48-44-40-36-32-28-24-20-16-12-8-4;/h5-48H2,1-4H3;1H/q+1;/p-1 | |
Source | PubChem | |
URL | https://pubchem.ncbi.nlm.nih.gov | |
Description | Data deposited in or computed by PubChem | |
InChI Key |
SMEFTBPJZGVAPK-UHFFFAOYSA-M | |
Source | PubChem | |
URL | https://pubchem.ncbi.nlm.nih.gov | |
Description | Data deposited in or computed by PubChem | |
Canonical SMILES |
CCCCCCCCCCCC[N+](CCCCCCCCCCCC)(CCCCCCCCCCCC)CCCCCCCCCCCC.[Br-] | |
Source | PubChem | |
URL | https://pubchem.ncbi.nlm.nih.gov | |
Description | Data deposited in or computed by PubChem | |
Molecular Formula |
C48H100BrN | |
Source | PubChem | |
URL | https://pubchem.ncbi.nlm.nih.gov | |
Description | Data deposited in or computed by PubChem | |
DSSTOX Substance ID |
DTXSID40933444 | |
Record name | N,N,N-Tridodecyldodecan-1-aminium bromide | |
Source | EPA DSSTox | |
URL | https://comptox.epa.gov/dashboard/DTXSID40933444 | |
Description | DSSTox provides a high quality public chemistry resource for supporting improved predictive toxicology. | |
Molecular Weight |
771.2 g/mol | |
Source | PubChem | |
URL | https://pubchem.ncbi.nlm.nih.gov | |
Description | Data deposited in or computed by PubChem | |
CAS No. |
14866-34-3 | |
Record name | Tetradodecylammonium bromide | |
Source | CAS Common Chemistry | |
URL | https://commonchemistry.cas.org/detail?cas_rn=14866-34-3 | |
Description | CAS Common Chemistry is an open community resource for accessing chemical information. Nearly 500,000 chemical substances from CAS REGISTRY cover areas of community interest, including common and frequently regulated chemicals, and those relevant to high school and undergraduate chemistry classes. This chemical information, curated by our expert scientists, is provided in alignment with our mission as a division of the American Chemical Society. | |
Explanation | The data from CAS Common Chemistry is provided under a CC-BY-NC 4.0 license, unless otherwise stated. | |
Record name | Tetradodecylammonium bromide | |
Source | ChemIDplus | |
URL | https://pubchem.ncbi.nlm.nih.gov/substance/?source=chemidplus&sourceid=0014866343 | |
Description | ChemIDplus is a free, web search system that provides access to the structure and nomenclature authority files used for the identification of chemical substances cited in National Library of Medicine (NLM) databases, including the TOXNET system. | |
Record name | N,N,N-Tridodecyldodecan-1-aminium bromide | |
Source | EPA DSSTox | |
URL | https://comptox.epa.gov/dashboard/DTXSID40933444 | |
Description | DSSTox provides a high quality public chemistry resource for supporting improved predictive toxicology. | |
Record name | Tetradodecylammonium bromide | |
Source | European Chemicals Agency (ECHA) | |
URL | https://echa.europa.eu/substance-information/-/substanceinfo/100.035.383 | |
Description | The European Chemicals Agency (ECHA) is an agency of the European Union which is the driving force among regulatory authorities in implementing the EU's groundbreaking chemicals legislation for the benefit of human health and the environment as well as for innovation and competitiveness. | |
Explanation | Use of the information, documents and data from the ECHA website is subject to the terms and conditions of this Legal Notice, and subject to other binding limitations provided for under applicable law, the information, documents and data made available on the ECHA website may be reproduced, distributed and/or used, totally or in part, for non-commercial purposes provided that ECHA is acknowledged as the source: "Source: European Chemicals Agency, http://echa.europa.eu/". Such acknowledgement must be included in each copy of the material. ECHA permits and encourages organisations and individuals to create links to the ECHA website under the following cumulative conditions: Links can only be made to webpages that provide a link to the Legal Notice page. | |
Q1: How does Tetradodecylammonium Bromide (TDAB) interact with perovskite materials in solar cell applications?
A1: TDAB acts as a post-surface modifier for perovskite films used in solar cells. It binds to the perovskite through hydrogen bonding, as confirmed by X-ray Photoelectron Spectroscopy (XPS) and Fourier Transform Infrared Spectroscopy (FTIR) analyses. [] This interaction leads to the formation of a (TDDA)2PbI1.66Br2.34 capping layer on the perovskite surface, effectively passivating defects. []
Q2: What is the impact of TDAB modification on the performance of perovskite solar cells?
A2: TDAB modification significantly enhances the power conversion efficiency (PCE) of perovskite solar cells. This improvement stems from the suppression of both electron and hole defects within the perovskite film, facilitating more efficient charge transport. [] For instance, a study reported a champion PCE of 21.33% for a TDAB-modified device. []
Q3: Beyond efficiency, how does TDAB treatment impact the stability of perovskite solar cells?
A3: The bulky cation of TDAB forms a hydrophobic capping layer on the perovskite surface, evidenced by a water contact angle of 93.39°. [] This hydrophobic layer acts as a barrier against moisture, a known degradation factor for perovskite materials. As a result, TDAB-modified PSCs exhibit remarkable stability, retaining their performance even after 30 days in humid air conditions (RH ≈ 40%). []
Q4: What is the role of TDAB in dispersing graphene sheets?
A4: TDAB, alongside other quaternary ammonium salts, can facilitate the dispersion of graphene oxide (GO) and reduced graphene oxide (RGO) in organic solvents like chloroform. [] This process relies on ionic interactions. The negatively charged carboxylic groups (COO-) on the GO or RGO surface interact with the positively charged ammonium group (NR4+) of TDAB. []
Q5: Does the alkyl chain length in quaternary ammonium salts influence their effectiveness in dispersing graphene sheets?
A5: Yes, the length of the alkyl chain significantly affects the effectiveness of quaternary ammonium salts in dispersing graphene. Studies comparing tetradecyltrimethylammonium bromide (TTAB), didodecyldimethylammonium bromide (DDAB), and TDAB (with 1, 2, and 4 alkyl chains, respectively) found DDAB to be the most effective. [] The longer chains in TDAB likely hinder efficient ionic interaction with GO or RGO due to steric effects. []
Q6: How is TDAB used in the development of taste sensors?
A6: TDAB serves as a lipid component in artificial lipid membranes for taste sensing applications. [] These membranes, often composed of TDAB, a plasticizer like dioctylphenyl phosphonate (DOPP), and a supporting polymer like polyvinyl chloride (PVC), act as the recognition element in the sensor. [] They transform chemical information from taste substances into measurable electrical potential changes. []
Q7: Can you provide details about the molecular formula, weight, and any relevant spectroscopic data for TDAB?
A7: The molecular formula for TDAB is C40H84BrN. It has a molecular weight of 667.01 g/mol. While the provided research doesn't include detailed spectroscopic data for TDAB, techniques like Fourier Transform Infrared Spectroscopy (FTIR) [] and X-ray Photoelectron Spectroscopy (XPS) [] have been used to characterize its interactions with other materials.
Q8: Are there any studies investigating the use of TDAB in ion-selective electrodes?
A8: Yes, research indicates that TDAB can be incorporated into ion-selective electrodes (ISEs). It has shown promise as an anion exchanger in nitrate ion-selective electrodes. [, ] In these applications, TDAB, along with a plasticizer like 2-nitrophenyl octyl ether, is incorporated into a PVC matrix to form the sensing membrane. []
Q9: Has TDAB been used in the synthesis of gold nanomaterials?
A9: Yes, TDAB plays a crucial role in the electrochemical synthesis of gold nanostructures. It acts as a stabilizer and micelle template, influencing the size and shape of the resulting nanoparticles. [, , , ] By adjusting the concentration of TDAB and other synthesis parameters, researchers have successfully fabricated gold nanocubes [] and gold nanodendrites. []
Q10: How does the concentration of TDAB affect the size of gold nanoparticles synthesized electrochemically?
A10: Research indicates an inverse relationship between TDAB concentration and the size of gold nanoparticles produced electrochemically. As the amount of TTAB surfactant increases, the size of the gold nanoparticles tends to decrease. []
Q11: What types of analytical techniques are used to characterize TDAB and its effects in various applications?
A11: Numerous analytical techniques have been employed to study TDAB, including:
- Surface characterization: X-ray photoelectron spectroscopy (XPS) [, ], Fourier transform infrared spectroscopy (FTIR) [, ], contact angle measurements [], surface zeta potential measurements [], gas cluster ion beam time-of-flight secondary ion mass spectrometry (GCIB-TOF-SIMS). []
- Material characterization: X-ray diffraction (XRD) [, , ], two-dimensional grazing incidence wide-angle X-ray scattering (2D GIWAXS) [], electron diffraction (ED) analysis [], transmission electron microscopy (TEM) [, ], ultraviolet-visible spectrometry (UV-Vis) [, , , , ].
- Electrochemical characterization: Cyclic voltammetry (CV) [], open circuit potential (OCP) measurements [].
Disclaimer and Information on In-Vitro Research Products
Please be aware that all articles and product information presented on BenchChem are intended solely for informational purposes. The products available for purchase on BenchChem are specifically designed for in-vitro studies, which are conducted outside of living organisms. In-vitro studies, derived from the Latin term "in glass," involve experiments performed in controlled laboratory settings using cells or tissues. It is important to note that these products are not categorized as medicines or drugs, and they have not received approval from the FDA for the prevention, treatment, or cure of any medical condition, ailment, or disease. We must emphasize that any form of bodily introduction of these products into humans or animals is strictly prohibited by law. It is essential to adhere to these guidelines to ensure compliance with legal and ethical standards in research and experimentation.