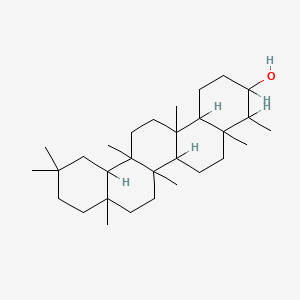
Friedelanol
Overview
Description
Friedelinol is a natural product found in Peritassa campestris, Eschenbachia blinii, and other organisms with data available.
Scientific Research Applications
Antiviral Properties:
- Friedelanol exhibits significant anti-Newcastle Disease Virus (NDV) activity. In a study, treatment with 3β-Friedelanol reduced the viral load to zero in embryonated chicken eggs, suggesting its potential as an anti-NDV agent (C. D., 2022).
- Another study reported the anti-human coronavirus (HCoV) activity of 3β-Friedelanol, highlighting its potential as a scaffold for developing new anti-HCoV drugs (F. Chang et al., 2012).
Antinociceptive Activity:
- Friedelanol and its derivatives have shown potent antinociceptive effects. For instance, a derivative, 3,15-dioxo-21α-hydroxy friedelane, exhibited significant dose-dependent effects in a study, indicating its potential for pain management (R. Niero et al., 2006).
Antimicrobial and Anti-Inflammatory Properties:
- Research has identified antimicrobial and anti-inflammatory properties in compounds related to Friedelanol. For instance, Friedelanol itself and its related compounds have been isolated from various plant species and evaluated for their potential in treating infections and inflammation (M. Duwiejua et al., 1999).
Cytotoxicity Against Carcinoma Cells:
- Friedelanol has been evaluated for its cytotoxic effects against human carcinoma cell lines. Studies indicate its potential role in the development of novel antiproliferative drugs (V. Kuete et al., 2017).
Pharmacological Properties in Traditional Medicine:
- Friedelanol has been identified in various medicinal plants and explored for its traditional therapeutic applications. It's often associated with anti-inflammatory and antimicrobial properties, reinforcing its potential use in traditional medicine (A. Sultana et al., 2022).
properties
IUPAC Name |
4,4a,6a,6b,8a,11,11,14a-octamethyl-1,2,3,4,5,6,6a,7,8,9,10,12,12a,13,14,14b-hexadecahydropicen-3-ol | |
---|---|---|
Details | Computed by Lexichem TK 2.7.0 (PubChem release 2021.05.07) | |
Source | PubChem | |
URL | https://pubchem.ncbi.nlm.nih.gov | |
Description | Data deposited in or computed by PubChem | |
InChI |
InChI=1S/C30H52O/c1-20-21(31)9-10-22-27(20,5)12-11-23-28(22,6)16-18-30(8)24-19-25(2,3)13-14-26(24,4)15-17-29(23,30)7/h20-24,31H,9-19H2,1-8H3 | |
Details | Computed by InChI 1.0.6 (PubChem release 2021.05.07) | |
Source | PubChem | |
URL | https://pubchem.ncbi.nlm.nih.gov | |
Description | Data deposited in or computed by PubChem | |
InChI Key |
XCDQFROEGGNAER-UHFFFAOYSA-N | |
Details | Computed by InChI 1.0.6 (PubChem release 2021.05.07) | |
Source | PubChem | |
URL | https://pubchem.ncbi.nlm.nih.gov | |
Description | Data deposited in or computed by PubChem | |
Canonical SMILES |
CC1C(CCC2C1(CCC3C2(CCC4(C3(CCC5(C4CC(CC5)(C)C)C)C)C)C)C)O | |
Details | Computed by OEChem 2.3.0 (PubChem release 2021.05.07) | |
Source | PubChem | |
URL | https://pubchem.ncbi.nlm.nih.gov | |
Description | Data deposited in or computed by PubChem | |
Molecular Formula |
C30H52O | |
Details | Computed by PubChem 2.1 (PubChem release 2021.05.07) | |
Source | PubChem | |
URL | https://pubchem.ncbi.nlm.nih.gov | |
Description | Data deposited in or computed by PubChem | |
Molecular Weight |
428.7 g/mol | |
Details | Computed by PubChem 2.1 (PubChem release 2021.05.07) | |
Source | PubChem | |
URL | https://pubchem.ncbi.nlm.nih.gov | |
Description | Data deposited in or computed by PubChem | |
Product Name |
Friedelinol | |
CAS RN |
5085-72-3 | |
Record name | Friedelanol | |
Source | DTP/NCI | |
URL | https://dtp.cancer.gov/dtpstandard/servlet/dwindex?searchtype=NSC&outputformat=html&searchlist=407041 | |
Description | The NCI Development Therapeutics Program (DTP) provides services and resources to the academic and private-sector research communities worldwide to facilitate the discovery and development of new cancer therapeutic agents. | |
Explanation | Unless otherwise indicated, all text within NCI products is free of copyright and may be reused without our permission. Credit the National Cancer Institute as the source. | |
Retrosynthesis Analysis
AI-Powered Synthesis Planning: Our tool employs the Template_relevance Pistachio, Template_relevance Bkms_metabolic, Template_relevance Pistachio_ringbreaker, Template_relevance Reaxys, Template_relevance Reaxys_biocatalysis model, leveraging a vast database of chemical reactions to predict feasible synthetic routes.
One-Step Synthesis Focus: Specifically designed for one-step synthesis, it provides concise and direct routes for your target compounds, streamlining the synthesis process.
Accurate Predictions: Utilizing the extensive PISTACHIO, BKMS_METABOLIC, PISTACHIO_RINGBREAKER, REAXYS, REAXYS_BIOCATALYSIS database, our tool offers high-accuracy predictions, reflecting the latest in chemical research and data.
Strategy Settings
Precursor scoring | Relevance Heuristic |
---|---|
Min. plausibility | 0.01 |
Model | Template_relevance |
Template Set | Pistachio/Bkms_metabolic/Pistachio_ringbreaker/Reaxys/Reaxys_biocatalysis |
Top-N result to add to graph | 6 |
Feasible Synthetic Routes
Disclaimer and Information on In-Vitro Research Products
Please be aware that all articles and product information presented on BenchChem are intended solely for informational purposes. The products available for purchase on BenchChem are specifically designed for in-vitro studies, which are conducted outside of living organisms. In-vitro studies, derived from the Latin term "in glass," involve experiments performed in controlled laboratory settings using cells or tissues. It is important to note that these products are not categorized as medicines or drugs, and they have not received approval from the FDA for the prevention, treatment, or cure of any medical condition, ailment, or disease. We must emphasize that any form of bodily introduction of these products into humans or animals is strictly prohibited by law. It is essential to adhere to these guidelines to ensure compliance with legal and ethical standards in research and experimentation.