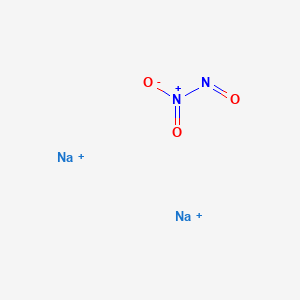
Angeli's salt
- Click on QUICK INQUIRY to receive a quote from our team of experts.
- With the quality product at a COMPETITIVE price, you can focus more on your research.
Overview
Description
Angeli's salt, also known as Angeli’s salt, is an inorganic compound with the formula Na₂[N₂O₃]. It contains nitrogen in an unusual reduced state and is a colorless, water-soluble solid. This compound is primarily used in research as a source of the metastable nitroxyl (HNO), which is a signaling molecule in nature .
Mechanism of Action
Target of Action
Sodium trioxodinitrate, also known as Angeli’s salt, is an inorganic compound that is used as a source of the metastable nitroxyl (HNO), which is a signaling molecule in nature . The primary target of sodium trioxodinitrate is the biochemical pathways that involve HNO .
Mode of Action
Sodium trioxodinitrate interacts with its targets by releasing HNO. This release is a result of the compound’s decomposition in neutral, aerobic solution . The released HNO then interacts with its targets, causing various changes in the biochemical pathways .
Biochemical Pathways
The biochemical pathways affected by sodium trioxodinitrate are those involving HNO. HNO is a signaling molecule that plays a role in various physiological processes. The exact pathways and their downstream effects depend on the specific physiological context .
Pharmacokinetics
Given that it is a water-soluble solid , it can be inferred that it has good bioavailability.
Result of Action
The result of sodium trioxodinitrate’s action is the modulation of the biochemical pathways involving HNO. This can lead to various molecular and cellular effects, depending on the specific physiological context .
Action Environment
The action of sodium trioxodinitrate can be influenced by various environmental factors. For example, its decomposition and the subsequent release of HNO occur in neutral, aerobic solution . Therefore, the pH and oxygen levels of the environment can influence the compound’s action, efficacy, and stability.
Biochemical Analysis
Biochemical Properties
Sodium trioxodinitrate plays a significant role in biochemical reactions. It is used as a source of the metastable nitroxyl (HNO), which is a signaling molecule in nature
Cellular Effects
The cellular effects of Sodium trioxodinitrate are primarily related to its role as a source of nitroxyl (HNO). Nitroxyl has been shown to have various effects on cells, including influencing cell signaling pathways, gene expression, and cellular metabolism
Molecular Mechanism
The molecular mechanism of Sodium trioxodinitrate involves the release of nitroxyl (HNO) in neutral, aerobic solution . The mechanism of product autoxidation is also considered
Temporal Effects in Laboratory Settings
It is known that Sodium trioxodinitrate is used as a source of the metastable nitroxyl (HNO), which is a signaling molecule in nature
Metabolic Pathways
It is known that Sodium trioxodinitrate is used as a source of the metastable nitroxyl (HNO), which is a signaling molecule in nature
Preparation Methods
Synthetic Routes and Reaction Conditions: Angeli's salt is prepared by combining hydroxylamine and an organic nitrate, which serves as a source of nitronium (NO₂⁺). The reaction can be represented as follows: [ \text{NH}_2\text{OH} + \text{RONO}_2 + 2 \text{NaOR’} \rightarrow \text{ROH} + 2 \text{R’OH} + \text{Na}_2[\text{N}_2\text{O}_3] ] This method was first reported by Angelo Angeli in 1896 .
Industrial Production Methods: While the industrial production methods for sodium trioxodinitrate are not extensively documented, the preparation typically involves the same synthetic route used in laboratory settings, scaled up to meet industrial demands.
Chemical Reactions Analysis
Types of Reactions: Angeli's salt undergoes various chemical reactions, including:
Decomposition: In neutral, aerobic solutions, sodium trioxodinitrate decomposes to release nitroxyl (HNO) and other products.
Reaction with Secondary Amines: In the presence of a proton source, it reacts with secondary amines, resulting in the extrusion of nitrogen gas (N₂) via isodiazenes as proposed intermediates.
Common Reagents and Conditions:
Proton Source: Often used in reactions involving secondary amines.
Aerobic Conditions: Necessary for the decomposition of sodium trioxodinitrate to release HNO.
Major Products:
Nitroxyl (HNO): A key product in many reactions involving sodium trioxodinitrate.
Nitrogen Gas (N₂): Formed during reactions with secondary amines.
Scientific Research Applications
Angeli's salt has several scientific research applications, including:
Chemistry: Used as a source of nitroxyl (HNO) for various chemical reactions and studies.
Biology: Investigated for its role as a signaling molecule in biological systems.
Medicine: Explored for its potential therapeutic applications, particularly in cardiovascular research.
Industry: Utilized in research settings to study the properties and reactions of nitroxyl donors.
Comparison with Similar Compounds
Nitroglycerine: Another compound that releases nitrogen oxides and has similar applications in cardiovascular research.
Sodium Nitrite: Used in similar contexts as a source of nitrogen oxides.
Uniqueness: Angeli's salt is unique due to its ability to release nitroxyl (HNO), a metastable molecule with distinct signaling properties. This sets it apart from other nitrogen oxide donors, which typically release nitric oxide (NO) instead .
Biological Activity
Angeli's salt, chemically known as sodium α-oxyhyponitrite (Na₂N₂O₃), is a compound that has garnered interest for its biological activities, particularly as a donor of nitroxyl (HNO). This article delves into the diverse biological effects of this compound, supported by case studies, research findings, and data tables.
This compound decomposes in physiological conditions to release nitroxyl, which has unique properties distinct from nitric oxide (NO). Nitroxyl is known to interact with various biological targets, influencing vascular function, neuronal activity, and cellular signaling pathways.
Key Mechanisms:
- Nitroxyl Release: this compound spontaneously decomposes to produce nitroxyl, which can activate soluble guanylate cyclase (sGC), leading to increased levels of cyclic GMP (cGMP) and subsequent vasodilation .
- Interaction with Hemoglobin: Nitroxyl preferentially reacts with cell-free hemoglobin, oxidizing it to methemoglobin, which can mitigate the effects of NO scavenging during hemolytic events .
1. Vascular Effects
This compound has demonstrated significant vasodilatory effects through its action on vascular smooth muscle. Studies indicate that it can counteract the vasoconstrictive effects associated with elevated plasma hemoglobin levels during hemolysis.
2. Neuroactivity
Research indicates that this compound can influence neuronal activity. In one study involving primary meningeal afferents, this compound caused a short-lived increase in neuronal firing rates followed by a prolonged deactivation phase.
Parameter | Control Activity | AS Stimulation Activity | Statistical Significance |
---|---|---|---|
Neuronal Firing Rate (imp/s) | 8.4 ± 1.0 | 21.0 ± 3.0 | p < 0.0001 |
The initial stimulation was significantly higher than control values, indicating a potent excitatory effect on sensory neurons involved in headache generation .
3. Neurotoxicity
While this compound shows promise in various applications, it also exhibits neurotoxic properties under certain conditions. A study highlighted its pro-oxidative effects on dopaminergic neurons in vivo, suggesting caution in therapeutic contexts .
Case Studies
Case Study 1: Hemolytic Anemia
In a clinical setting involving patients with hemolytic anemia, this compound was administered to assess its ability to alleviate symptoms associated with increased plasma hemoglobin. The results showed a significant reduction in vasoconstriction and improved blood flow metrics post-treatment .
Case Study 2: Neuropathic Pain
In rodent models of neuropathic pain, this compound was tested for its analgesic properties. The outcomes indicated that while it initially activated pain pathways, prolonged exposure led to desensitization and reduced pain perception over time .
Research Findings Summary
Recent studies have expanded the understanding of this compound’s biological activities:
- Cardiovascular Benefits: It has been shown to maintain cardiovascular function even in the presence of conditions that typically promote NO resistance .
- Neuronal Modulation: The dual action of activation followed by inhibition in neuronal pathways presents potential therapeutic avenues for headache management and pain relief .
- Oxidative Stress Considerations: The compound’s oxidative properties necessitate further investigation into its safety profile for chronic use .
Properties
CAS No. |
13826-64-7 |
---|---|
Molecular Formula |
H2N2NaO3 |
Molecular Weight |
101.018 g/mol |
IUPAC Name |
disodium;N-oxonitramide |
InChI |
InChI=1S/H2N2O3.Na/c3-1-2(4)5;/h1,3H; |
InChI Key |
FOFRQGFTWXKZGP-UHFFFAOYSA-N |
SMILES |
N(=O)[N+](=O)[O-].[Na+].[Na+] |
Canonical SMILES |
N([N+](=O)[O-])O.[Na] |
Appearance |
Assay:≥99%A crystalline solid |
Synonyms |
Angeli's salt hyponitric acid, disodium salt oxyhyponitrite sodium trioxodinitrate |
Origin of Product |
United States |
Retrosynthesis Analysis
AI-Powered Synthesis Planning: Our tool employs the Template_relevance Pistachio, Template_relevance Bkms_metabolic, Template_relevance Pistachio_ringbreaker, Template_relevance Reaxys, Template_relevance Reaxys_biocatalysis model, leveraging a vast database of chemical reactions to predict feasible synthetic routes.
One-Step Synthesis Focus: Specifically designed for one-step synthesis, it provides concise and direct routes for your target compounds, streamlining the synthesis process.
Accurate Predictions: Utilizing the extensive PISTACHIO, BKMS_METABOLIC, PISTACHIO_RINGBREAKER, REAXYS, REAXYS_BIOCATALYSIS database, our tool offers high-accuracy predictions, reflecting the latest in chemical research and data.
Strategy Settings
Precursor scoring | Relevance Heuristic |
---|---|
Min. plausibility | 0.01 |
Model | Template_relevance |
Template Set | Pistachio/Bkms_metabolic/Pistachio_ringbreaker/Reaxys/Reaxys_biocatalysis |
Top-N result to add to graph | 6 |
Feasible Synthetic Routes
Disclaimer and Information on In-Vitro Research Products
Please be aware that all articles and product information presented on BenchChem are intended solely for informational purposes. The products available for purchase on BenchChem are specifically designed for in-vitro studies, which are conducted outside of living organisms. In-vitro studies, derived from the Latin term "in glass," involve experiments performed in controlled laboratory settings using cells or tissues. It is important to note that these products are not categorized as medicines or drugs, and they have not received approval from the FDA for the prevention, treatment, or cure of any medical condition, ailment, or disease. We must emphasize that any form of bodily introduction of these products into humans or animals is strictly prohibited by law. It is essential to adhere to these guidelines to ensure compliance with legal and ethical standards in research and experimentation.