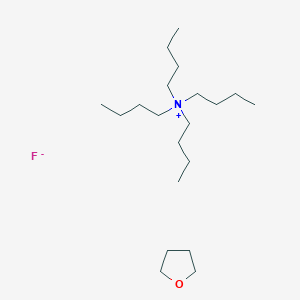
TBAF tetrahydrofuran
Overview
Description
Tetrabutylammonium fluoride in tetrahydrofuran is a quaternary ammonium salt with the chemical formula (C₄H₉)₄NF. It is commonly used as a source of fluoride ion in organic solvents. This compound is commercially available as a white solid trihydrate and as a solution in tetrahydrofuran. Tetrabutylammonium fluoride in tetrahydrofuran is known for its ability to act as a strong hydrogen bond acceptor, making it highly useful in various chemical reactions .
Preparation Methods
Tetrabutylammonium fluoride in tetrahydrofuran can be prepared by passing hydrofluoric acid through an ion-exchange resin, followed by tetrabutylammonium bromide. Upon evaporation of the water, tetrabutylammonium fluoride can be collected as an oil in quantitative yield . Another method involves dissolving water-containing tetrabutylammonium fluoride in an organic solvent, carrying out azeotropic water-carrying through a water separator, then evaporating the solvent, and finally cooling to separate out the anhydrous tetrabutylammonium fluoride .
Chemical Reactions Analysis
Tetrabutylammonium fluoride in tetrahydrofuran undergoes various types of chemical reactions, including:
Deprotection Reactions: It is used to remove silyl ether protecting groups in organic synthesis.
Fluorination Reactions: It serves as a reagent in fluorination reactions.
Cyclization Reactions: It is used to synthesize 2-substituted indoles by cyclization reaction of various 2-ethynylanilines with terminal alkynes using palladium catalyst.
Coupling Reactions: It acts as an activator in the synthesis of arylated or alkenylated alkynes by the coupling reaction of aryl and alkenyl halides with terminal alkynes in the presence of palladium catalyst.
Scientific Research Applications
N-Alkylation Reactions
TBAF has been shown to significantly accelerate N-alkylation reactions, particularly involving purine derivatives. In a study, TBAF facilitated the N9-alkylation of purine rings with various organic halides at room temperature, achieving high yields within minutes. This method allows for rapid synthesis of purine libraries, which are crucial for drug discovery and enzyme inhibition studies .
Reaction Type | Conditions | Yield | Time |
---|---|---|---|
N9-Alkylation of Purines | TBAF (2 equiv), RT | High | 10 min |
TBAF acts as an efficient catalyst for the addition of trialkylsilylalkynes to aldehydes and ketones in THF at room temperature. This method tolerates various functional groups and generally provides better yields compared to traditional methods, making it a valuable tool in synthetic organic chemistry .
Reaction Type | Substrate | Yield |
---|---|---|
Addition to Aldehydes | Trialkylsilylalkynes | Comparable or better than literature |
Synthesis of Complex Molecules
In the synthesis of complex biomolecules, such as halichondrin derivatives, TBAF has been employed for its efficiency in promoting key transformations while simplifying purification processes. The combination of TBAF with ion-exchange resins has proven effective in removing residual salts and enhancing product yields .
Case Study 1: Rapid Synthesis of Purine Libraries
A study published in Organic Letters demonstrated the use of TBAF for the rapid synthesis of N9-alkylated purine analogues. The researchers reported that using TBAF allowed them to complete reactions within 10 minutes with excellent selectivity between N9 and N7 alkylation products, paving the way for high-throughput screening applications .
Case Study 2: Efficient Work-Up Procedures
Another significant advancement was made regarding the work-up procedures following TBAF-mediated reactions. Researchers developed a method combining sulfonic acid resins with calcium carbonate to effectively remove excess TBAF from reaction mixtures, achieving over 98% removal efficiency while maintaining product integrity .
Mechanism of Action
The mechanism of action of tetrabutylammonium fluoride in tetrahydrofuran involves its ability to act as a strong hydrogen bond acceptor. This property allows it to effectively remove silyl ether protecting groups and participate in various chemical reactions. The fluoride ion in tetrabutylammonium fluoride is highly reactive and can form strong bonds with electrophiles, facilitating various organic transformations .
Comparison with Similar Compounds
Tetrabutylammonium fluoride in tetrahydrofuran is unique due to its strong hydrogen bond accepting ability and its solubility in organic solvents. Similar compounds include:
Tetramethylammonium fluoride: Another quaternary ammonium salt used as a fluoride source.
Tetraethylammonium fluoride: Similar in structure but with ethyl groups instead of butyl groups.
Tetrabutylammonium bromide: Used in the preparation of tetrabutylammonium fluoride.
Tetrabutylammonium fluoride in tetrahydrofuran stands out due to its higher solubility in organic solvents and its effectiveness in deprotection and fluorination reactions.
Q & A
Basic Research Questions
Q. What are the critical considerations for handling and storing TBAF in tetrahydrofuran (THF) solutions to ensure reagent stability and reaction reproducibility?
TBAF in THF is highly sensitive to moisture and atmospheric conditions. Solutions should be stored under anhydrous conditions at recommended temperatures (e.g., 2–8°C) and used promptly after opening to avoid hydrolysis or decomposition. The presence of water in TBAF/THF solutions can significantly alter reactivity, as seen in deprotection reactions where excess water (>10 equiv) quenches basicity, leading to incomplete reactions or side-product formation . Pre-drying solvents (e.g., THF over Na/K alloy) and using molecular sieves during reactions can mitigate moisture interference .
Q. What is the standard protocol for TBAF-mediated deprotection of silyl ethers, and how do reaction conditions influence efficiency?
TBAF (1 M in THF) is typically added stoichiometrically (1–2 equiv) to silyl-protected substrates at room temperature. Reaction times vary (minutes to hours) depending on steric hindrance and silyl group stability (e.g., TBS vs. TIPS). For example, deprotection of a TBS group in a sterically hindered environment required 2 hours at 20°C, yielding 84% product . However, excessive TBAF or prolonged reaction times can lead to side reactions, such as cyclization or over-oxidation . Monitoring via TLC or NMR is critical to avoid degradation.
Advanced Research Questions
Q. How can researchers address low yields and side-product formation during TBAF-mediated deprotection of complex substrates?
Competing pathways, such as premature cyclization or acid-sensitive group degradation, often arise due to TBAF’s strong basicity. A two-step approach can improve yields: (1) Controlled addition of water (≤10 equiv) to attenuate TBAF’s reactivity, followed by (2) molecular sieve addition to sequester water and activate residual TBAF for secondary deprotection steps. This method increased yields from 12% to 39% in a dual Teoc/TBS deprotection . Alternatively, using buffered TBAF systems (e.g., with AcOH) can modulate basicity for sensitive substrates .
Q. How does TBAF enable one-pot deprotection and subsequent transformations, such as Michael additions or cyclizations?
TBAF’s dual role as a deprotecting agent and mild base allows sequential reactions in a single pot. For example, after deprotecting a silyl ether, excess TBAF (2 equiv) facilitated an intramolecular Michael addition, yielding a cyclized product in 77% yield . Key parameters include maintaining anhydrous conditions during deprotection and optimizing TBAF stoichiometry to avoid over-basification, which could degrade intermediates .
Q. What mechanistic insights support TBAF’s role in facilitating double C–H functionalization for tetrahydrofuran ring formation?
TBAF/air systems promote diastereoselective C–H oxidation and subsequent ether linkage formation. In the synthesis of welwitindolinone alkaloids, TBAF likely deprotonates intermediates, enabling oxygen insertion via radical or electrophilic pathways. This method achieved tetrahydrofuran ring formation in a single step, outperforming traditional bases like K₂CO₃ . Reaction monitoring via ³¹P NMR or LC-MS is essential to track intermediate stability and oxidation states .
Q. How does TBAF enhance semiclathrate formation for CO₂ capture, and what are the implications for gas hydrate studies?
TBAF acts as a thermodynamic promoter in semiclathrate hydrates, integrating into the host framework and stabilizing CO₂ in small cages. Unlike THF, which only occupies large cages, TBAF’s quaternary ammonium structure lowers equilibrium pressure requirements for hydrate formation. Optimal TBAF concentrations (e.g., 19 wt%) and kinetic additives (e.g., SDS) improve CO₂ uptake efficiency . This has applications in carbon capture and energy storage technologies.
Q. What methodologies enable the use of TBAF in fluorine-18 (¹⁸F) labeling for radiopharmaceutical synthesis?
TBAF efficiently cleaves oxydithiaphospholane 2-sulfide substrates to generate fluorothiophosphate (FTP) synthons for ¹⁸F incorporation. Reactions in THF with excess TBAF achieve >95% conversion in 2 minutes, enabling rapid radiolabeling of biomolecules. Critical steps include substrate pre-oxidation with sulfur and rigorous solvent drying to minimize side reactions .
Q. How does TBAF-mediated deprotection influence the synthesis of catechol-functionalized polymers for underwater adhesives?
TBAF cleaves O-TBS protecting groups in polyacrylamide-based polymers, exposing catechol moieties for cross-linking. Immediate use post-deprotection is vital to prevent oxidation of catechol to quinones, which reduces adhesive strength. Optimal conditions involve 1.2 equiv TBAF in THF at 0°C for 10 minutes, followed by rapid solvent removal .
Q. Methodological Best Practices
- Reagent Purity : Verify TBAF/THF solution water content (e.g., ≤5% w/w) via Karl Fischer titration, as excess water reduces reactivity .
- Reaction Monitoring : Use real-time techniques like TLC or in-situ NMR to detect intermediates (e.g., tetrahydrofuran cyclization products) .
- Scale-Up Considerations : For large-scale deprotections, gradual TBAF addition and temperature control (−20°C to RT) prevent exothermic side reactions .
Properties
IUPAC Name |
oxolane;tetrabutylazanium;fluoride | |
---|---|---|
Details | Computed by LexiChem 2.6.6 (PubChem release 2019.06.18) | |
Source | PubChem | |
URL | https://pubchem.ncbi.nlm.nih.gov | |
Description | Data deposited in or computed by PubChem | |
InChI |
InChI=1S/C16H36N.C4H8O.FH/c1-5-9-13-17(14-10-6-2,15-11-7-3)16-12-8-4;1-2-4-5-3-1;/h5-16H2,1-4H3;1-4H2;1H/q+1;;/p-1 | |
Details | Computed by InChI 1.0.5 (PubChem release 2019.06.18) | |
Source | PubChem | |
URL | https://pubchem.ncbi.nlm.nih.gov | |
Description | Data deposited in or computed by PubChem | |
InChI Key |
YWWARDMVSMPOLR-UHFFFAOYSA-M | |
Details | Computed by InChI 1.0.5 (PubChem release 2019.06.18) | |
Source | PubChem | |
URL | https://pubchem.ncbi.nlm.nih.gov | |
Description | Data deposited in or computed by PubChem | |
Canonical SMILES |
CCCC[N+](CCCC)(CCCC)CCCC.C1CCOC1.[F-] | |
Details | Computed by OEChem 2.1.5 (PubChem release 2019.06.18) | |
Source | PubChem | |
URL | https://pubchem.ncbi.nlm.nih.gov | |
Description | Data deposited in or computed by PubChem | |
Molecular Formula |
C20H44FNO | |
Details | Computed by PubChem 2.1 (PubChem release 2019.06.18) | |
Source | PubChem | |
URL | https://pubchem.ncbi.nlm.nih.gov | |
Description | Data deposited in or computed by PubChem | |
Molecular Weight |
333.6 g/mol | |
Details | Computed by PubChem 2.1 (PubChem release 2021.05.07) | |
Source | PubChem | |
URL | https://pubchem.ncbi.nlm.nih.gov | |
Description | Data deposited in or computed by PubChem | |
Synthesis routes and methods
Procedure details
Retrosynthesis Analysis
AI-Powered Synthesis Planning: Our tool employs the Template_relevance Pistachio, Template_relevance Bkms_metabolic, Template_relevance Pistachio_ringbreaker, Template_relevance Reaxys, Template_relevance Reaxys_biocatalysis model, leveraging a vast database of chemical reactions to predict feasible synthetic routes.
One-Step Synthesis Focus: Specifically designed for one-step synthesis, it provides concise and direct routes for your target compounds, streamlining the synthesis process.
Accurate Predictions: Utilizing the extensive PISTACHIO, BKMS_METABOLIC, PISTACHIO_RINGBREAKER, REAXYS, REAXYS_BIOCATALYSIS database, our tool offers high-accuracy predictions, reflecting the latest in chemical research and data.
Strategy Settings
Precursor scoring | Relevance Heuristic |
---|---|
Min. plausibility | 0.01 |
Model | Template_relevance |
Template Set | Pistachio/Bkms_metabolic/Pistachio_ringbreaker/Reaxys/Reaxys_biocatalysis |
Top-N result to add to graph | 6 |
Feasible Synthetic Routes
Disclaimer and Information on In-Vitro Research Products
Please be aware that all articles and product information presented on BenchChem are intended solely for informational purposes. The products available for purchase on BenchChem are specifically designed for in-vitro studies, which are conducted outside of living organisms. In-vitro studies, derived from the Latin term "in glass," involve experiments performed in controlled laboratory settings using cells or tissues. It is important to note that these products are not categorized as medicines or drugs, and they have not received approval from the FDA for the prevention, treatment, or cure of any medical condition, ailment, or disease. We must emphasize that any form of bodily introduction of these products into humans or animals is strictly prohibited by law. It is essential to adhere to these guidelines to ensure compliance with legal and ethical standards in research and experimentation.