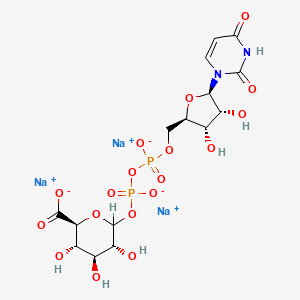
Udpga 3Na
Overview
Description
Preparation Methods
Synthetic Routes and Reaction Conditions
The synthesis of uridine 5’-diphosphoglucuronic acid trisodium salt can be achieved through a cascade synthesis route. This involves coupling multiple whole cells expressing hyperthermophilic enzymes to produce uridine 5’-diphosphoglucuronic acid from starch. The process includes a coenzyme regeneration system with an appropriate expression level of uridine diphosphate-glucose 6-dehydrogenase in a single strain, meeting the nicotinamide adenine dinucleotide requirements .
Industrial Production Methods
For industrial production, the cascade synthesis route is scaled up to produce uridine 5’-diphosphoglucuronic acid in large quantities. This method is cost-effective and efficient, yielding high purity uridine 5’-diphosphoglucuronic acid suitable for various applications .
Chemical Reactions Analysis
Types of Reactions
Uridine 5’-diphosphoglucuronic acid trisodium salt undergoes glucuronidation reactions, which are catalyzed by uridine diphosphate glucuronosyltransferases. These reactions involve the covalent linkage of glucuronic acid to a nucleophilic functional group on a typically lipophilic substrate .
Common Reagents and Conditions
The glucuronidation reactions require the presence of uridine 5’-diphosphoglucuronic acid as a cofactor and a nucleophilic substrate. The reactions typically proceed under physiological conditions, with the enzyme uridine diphosphate glucuronosyltransferase facilitating the transfer of glucuronic acid .
Major Products
The major products of these reactions are glucuronides, which are more water-soluble and can be easily excreted from the body. This process is essential for the metabolism and clearance of many lipophilic chemicals, including drugs and endogenous compounds .
Scientific Research Applications
Uridine 5’-diphosphoglucuronic acid trisodium salt has numerous applications in scientific research:
Mechanism of Action
Uridine 5’-diphosphoglucuronic acid trisodium salt exerts its effects through glucuronidation, a process catalyzed by uridine diphosphate glucuronosyltransferases. The enzyme facilitates the transfer of glucuronic acid to a nucleophilic functional group on a substrate, forming a glucuronide. This reaction increases the water solubility of the substrate, allowing for its excretion from the body .
Comparison with Similar Compounds
Uridine 5’-diphosphoglucuronic acid trisodium salt is unique in its role as a glycosyl donor for glucuronidation reactions. Similar compounds include:
Uridine diphosphate glucose: A precursor in the biosynthesis of uridine 5’-diphosphoglucuronic acid.
Uridine diphosphate galactose: Involved in the biosynthesis of glycoproteins and glycolipids.
Uridine diphosphate N-acetylglucosamine: Participates in the biosynthesis of glycosaminoglycans and glycoproteins.
Uridine 5’-diphosphoglucuronic acid trisodium salt stands out due to its specific role in glucuronidation, a critical pathway for the detoxification and excretion of various compounds .
Biological Activity
UDPGA, or uridine diphosphate glucuronic acid, is a crucial co-substrate in the glucuronidation pathway, primarily catalyzed by UDP-glucuronosyltransferases (UGTs). This article provides a comprehensive overview of the biological activity of UDPGA, focusing on its role in drug metabolism, transport mechanisms, and implications in various physiological and pathological contexts.
Overview of UDPGA
UDPGA is synthesized from UDP-glucose through the action of UDP-glucose dehydrogenase (UGD). It serves as a donor of glucuronic acid in the conjugation processes that facilitate the excretion of lipophilic compounds by converting them into more hydrophilic forms. This transformation enhances their solubility and promotes elimination via urine or bile.
Transport Mechanisms
The transport of UDPGA into the endoplasmic reticulum (ER) is essential for UGT activity. Recent studies have identified specific nucleotide sugar transporters, notably SLC35B1, as key players in this process. The knockdown of SLC35B1 significantly reduces UGT activity, indicating its pivotal role in transporting UDPGA into the ER for effective glucuronidation .
Table 1: Key Transporters Involved in UDPGA Transport
Transporter | Function | Impact on UGT Activity |
---|---|---|
SLC35B1 | Major transporter for UDPGA into ER | Significant decrease upon knockdown |
SLC35B4 | Minor transporter | Limited impact on UGT activity |
SLC35D1 | Contributes to UDPGA transport | Moderate variability in expression |
Role in Drug Metabolism
UDPGA's primary function is to facilitate the glucuronidation of drugs and other xenobiotics. UGTs catalyze the transfer of glucuronic acid from UDPGA to various substrates, enhancing their elimination. For instance, studies have shown that UGT1A1 and UGT2B7 are significantly involved in the metabolism of common pharmaceuticals such as paracetamol and morphine .
Case Study: Paracetamol Metabolism
A study investigating the metabolism of paracetamol revealed that genetic variations in UGT enzymes could lead to altered glucuronidation rates. Individuals with certain UGT polymorphisms exhibited reduced glucuronidation efficacy, resulting in higher plasma concentrations of paracetamol and increased risk for hepatotoxicity .
Implications in Cancer Biology
Research indicates that alterations in UGT expression and activity can influence tumor metabolism and progression. In cancer cells, particularly prostate cancer, UDPGA contributes to the synthesis of proteoglycans that may affect tumor growth and survival by modulating intracellular signaling pathways .
Table 2: Impact of UGT Activity on Tumor Metabolism
Tumor Type | Altered UGT Expression | Effect on Tumor Growth |
---|---|---|
Prostate Cancer | Increased UGT1A1 | Enhanced proteoglycan synthesis |
Breast Cancer | Decreased UGT2B7 | Impaired drug metabolism |
Regulatory Mechanisms
The expression and activity of UGTs are regulated by a variety of mechanisms including epigenetic modifications (e.g., DNA methylation), transcriptional regulation, and post-translational modifications. These regulatory pathways are crucial for maintaining homeostasis and can be disrupted in disease states such as cancer .
Properties
IUPAC Name |
trisodium;(2S,3S,4S,5R)-6-[[[(2R,3S,4R,5R)-5-(2,4-dioxopyrimidin-1-yl)-3,4-dihydroxyoxolan-2-yl]methoxy-oxidophosphoryl]oxy-oxidophosphoryl]oxy-3,4,5-trihydroxyoxane-2-carboxylate | |
---|---|---|
Details | Computed by Lexichem TK 2.7.0 (PubChem release 2021.05.07) | |
Source | PubChem | |
URL | https://pubchem.ncbi.nlm.nih.gov | |
Description | Data deposited in or computed by PubChem | |
InChI |
InChI=1S/C15H22N2O18P2.3Na/c18-5-1-2-17(15(26)16-5)12-9(22)6(19)4(32-12)3-31-36(27,28)35-37(29,30)34-14-10(23)7(20)8(21)11(33-14)13(24)25;;;/h1-2,4,6-12,14,19-23H,3H2,(H,24,25)(H,27,28)(H,29,30)(H,16,18,26);;;/q;3*+1/p-3/t4-,6-,7+,8+,9-,10-,11+,12-,14?;;;/m1.../s1 | |
Details | Computed by InChI 1.0.6 (PubChem release 2021.05.07) | |
Source | PubChem | |
URL | https://pubchem.ncbi.nlm.nih.gov | |
Description | Data deposited in or computed by PubChem | |
InChI Key |
XXXUNWUNTOMVIG-FBPUXPPNSA-K | |
Details | Computed by InChI 1.0.6 (PubChem release 2021.05.07) | |
Source | PubChem | |
URL | https://pubchem.ncbi.nlm.nih.gov | |
Description | Data deposited in or computed by PubChem | |
Canonical SMILES |
C1=CN(C(=O)NC1=O)C2C(C(C(O2)COP(=O)([O-])OP(=O)([O-])OC3C(C(C(C(O3)C(=O)[O-])O)O)O)O)O.[Na+].[Na+].[Na+] | |
Details | Computed by OEChem 2.3.0 (PubChem release 2021.05.07) | |
Source | PubChem | |
URL | https://pubchem.ncbi.nlm.nih.gov | |
Description | Data deposited in or computed by PubChem | |
Isomeric SMILES |
C1=CN(C(=O)NC1=O)[C@H]2[C@@H]([C@@H]([C@H](O2)COP(=O)([O-])OP(=O)([O-])OC3[C@@H]([C@H]([C@@H]([C@H](O3)C(=O)[O-])O)O)O)O)O.[Na+].[Na+].[Na+] | |
Details | Computed by OEChem 2.3.0 (PubChem release 2021.05.07) | |
Source | PubChem | |
URL | https://pubchem.ncbi.nlm.nih.gov | |
Description | Data deposited in or computed by PubChem | |
Molecular Formula |
C15H19N2Na3O18P2 | |
Details | Computed by PubChem 2.1 (PubChem release 2021.05.07) | |
Source | PubChem | |
URL | https://pubchem.ncbi.nlm.nih.gov | |
Description | Data deposited in or computed by PubChem | |
Molecular Weight |
646.23 g/mol | |
Details | Computed by PubChem 2.1 (PubChem release 2021.05.07) | |
Source | PubChem | |
URL | https://pubchem.ncbi.nlm.nih.gov | |
Description | Data deposited in or computed by PubChem | |
Retrosynthesis Analysis
AI-Powered Synthesis Planning: Our tool employs the Template_relevance Pistachio, Template_relevance Bkms_metabolic, Template_relevance Pistachio_ringbreaker, Template_relevance Reaxys, Template_relevance Reaxys_biocatalysis model, leveraging a vast database of chemical reactions to predict feasible synthetic routes.
One-Step Synthesis Focus: Specifically designed for one-step synthesis, it provides concise and direct routes for your target compounds, streamlining the synthesis process.
Accurate Predictions: Utilizing the extensive PISTACHIO, BKMS_METABOLIC, PISTACHIO_RINGBREAKER, REAXYS, REAXYS_BIOCATALYSIS database, our tool offers high-accuracy predictions, reflecting the latest in chemical research and data.
Strategy Settings
Precursor scoring | Relevance Heuristic |
---|---|
Min. plausibility | 0.01 |
Model | Template_relevance |
Template Set | Pistachio/Bkms_metabolic/Pistachio_ringbreaker/Reaxys/Reaxys_biocatalysis |
Top-N result to add to graph | 6 |
Feasible Synthetic Routes
Disclaimer and Information on In-Vitro Research Products
Please be aware that all articles and product information presented on BenchChem are intended solely for informational purposes. The products available for purchase on BenchChem are specifically designed for in-vitro studies, which are conducted outside of living organisms. In-vitro studies, derived from the Latin term "in glass," involve experiments performed in controlled laboratory settings using cells or tissues. It is important to note that these products are not categorized as medicines or drugs, and they have not received approval from the FDA for the prevention, treatment, or cure of any medical condition, ailment, or disease. We must emphasize that any form of bodily introduction of these products into humans or animals is strictly prohibited by law. It is essential to adhere to these guidelines to ensure compliance with legal and ethical standards in research and experimentation.