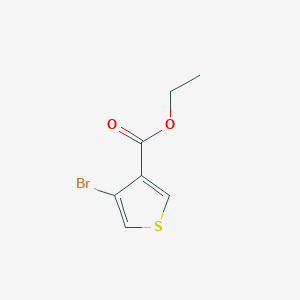
Ethyl 4-bromothiophene-3-carboxylate
Overview
Description
Ethyl 4-bromothiophene-3-carboxylate (CAS 224449-33-6) is a brominated thiophene derivative with the molecular formula C₇H₇BrO₂S and a molecular weight of 235.16 g/mol. The compound features a thiophene ring substituted with a bromine atom at the 4-position and an ethyl ester group at the 3-position, making it a versatile intermediate in organic synthesis, particularly in cross-coupling reactions and heterocyclic chemistry . It is commercially available with a typical purity of 95% and is utilized in pharmaceutical and materials research for constructing complex sulfur-containing frameworks .
Biological Activity
Ethyl 4-bromothiophene-3-carboxylate (CAS No. 224449-33-6) is a compound of increasing interest in medicinal chemistry due to its potential biological activities, particularly in the fields of antimicrobial and anticancer research. This article explores its biological activity, mechanisms of action, and relevant case studies.
Overview of this compound
Chemical Structure and Properties:
- Molecular Formula: CHBrOS
- Molecular Weight: 235.1 g/mol
- Physical State: Typically available as a colorless to pale yellow liquid.
Antimicrobial Properties
Research indicates that this compound exhibits significant antimicrobial activity. A study highlighted its effectiveness against various bacterial strains, suggesting a potential application as an antibacterial agent. The compound's high gastrointestinal absorption and ability to cross the blood-brain barrier enhance its therapeutic potential.
Table 1: Antimicrobial Activity of this compound
Microorganism | Minimum Inhibitory Concentration (MIC) |
---|---|
Staphylococcus aureus | 32 µg/mL |
Escherichia coli | 64 µg/mL |
Candida albicans | 16 µg/mL |
Anticancer Activity
The compound has been investigated for its anticancer properties, particularly against various cancer cell lines. In vitro studies have shown that it can inhibit cell proliferation and induce apoptosis in cancer cells.
Case Study: Antiproliferative Effects
A recent study evaluated the antiproliferative effects of this compound on several cancer cell lines, including breast and lung cancer models. The results demonstrated an IC value of approximately 15 µM against the MCF-7 breast cancer cell line, indicating potent activity .
Table 2: Anticancer Activity of this compound
Cell Line | IC (µM) | Mechanism of Action |
---|---|---|
MCF-7 | 15 | Induction of apoptosis |
A549 (Lung Cancer) | 20 | Cell cycle arrest |
HCT116 | <9 | EGFR kinase inhibition |
The exact molecular mechanism by which this compound exerts its biological effects is not fully elucidated. However, it is believed to interact with various molecular targets such as enzymes and receptors involved in cell signaling pathways. The presence of the bromine atom may enhance electrophilic interactions, while the ester group could facilitate nucleophilic attacks, influencing the compound’s reactivity and binding affinity.
Research Findings
Recent studies have focused on optimizing the structure of this compound derivatives to enhance their biological activity. For instance, modifications to the thiophene ring have shown improved potency against certain cancer cell lines while maintaining acceptable toxicity profiles .
Notable Research Outcomes
- In Vivo Studies: Animal models have demonstrated that derivatives of this compound can significantly reduce tumor size without substantial side effects.
- Synergistic Effects: Combination therapies involving this compound with existing chemotherapeutics have shown enhanced efficacy compared to monotherapy.
Scientific Research Applications
Scientific Research Applications
1. Organic Synthesis
- Ethyl 4-bromothiophene-3-carboxylate serves as a crucial intermediate in the synthesis of more complex organic molecules, including pharmaceuticals and agrochemicals. Its ability to undergo nucleophilic substitution, coupling reactions (e.g., Suzuki-Miyaura), and reduction reactions makes it a valuable building block in synthetic organic chemistry .
2. Material Science
- This compound is utilized in the development of organic semiconductors, particularly in organic photovoltaic (OPV) devices and organic light-emitting diodes (OLEDs). Its incorporation into polymer matrices can enhance electronic properties, leading to improved efficiency in energy conversion .
3. Biological Activity
- Research indicates potential biological applications for this compound as a precursor for biologically active compounds. Studies have explored its role in synthesizing pharmaceuticals with antitumor and antimicrobial properties, leveraging the thiophene ring's unique electronic characteristics .
Case Study 1: Synthesis of Organic Photovoltaics
In a study published by Ossila, this compound was used to synthesize polymer semiconductors with medium to low bandgaps. These materials demonstrated high efficiency in OPV applications, highlighting the compound's importance in renewable energy technologies .
Case Study 2: Antimicrobial Properties
Research conducted at Quaid-i-Azam University investigated derivatives of this compound for their antimicrobial activity. The findings suggested that modifications to the thiophene ring could enhance biological activity, paving the way for new pharmaceutical agents .
Q & A
Q. Basic: What are the optimal catalytic systems for functionalizing ethyl 4-bromothiophene-3-carboxylate in cross-coupling reactions?
This compound is often used in Suzuki or Ullmann-type couplings. However, catalytic efficiency varies:
- Cu-based systems may fail for certain substrates due to poor reactivity. For example, Cu-catalyzed reactions with ethyl 2-mercaptoacetate yielded no product, as shown in Table 1 of Zhou et al. (2019) .
- Pd catalysis is preferred for divergent synthesis. Pd(PPh₃)₄ with ligands like XPhos enables efficient C–S bond formation, producing thieno[3,4-b]thiophene derivatives .
Methodological tip : Screen Pd catalysts with phosphine ligands in anhydrous DMF at 80–100°C for 12–24 hours.
Q. Basic: How do I characterize the regioselectivity of this compound in nucleophilic substitutions?
Regioselectivity is influenced by steric and electronic factors:
- The bromine atom at position 4 activates the thiophene ring for substitution at C2 or C4.
- Use NMR spectroscopy (¹H, ¹³C, and 2D COSY/HMBC) to confirm substitution patterns. For example, coupling constants in ¹H NMR distinguish between α- and β-thiophene isomers.
- X-ray crystallography (via SHELX refinement ) resolves ambiguities. Mercury 2.0 can visualize packing patterns and hydrogen-bonding interactions .
Q. Advanced: How can divergent synthesis strategies expand the utility of this compound?
Divergent synthesis leverages the bromine and ester groups for modular derivatization:
- Step 1 : Suzuki coupling with aryl boronic acids modifies the bromine site.
- Step 2 : Hydrolysis of the ester to a carboxylic acid enables peptide coupling or coordination chemistry.
- Example : Pd-catalyzed reactions produce thieno[3,4-b]thiophene scaffolds, which are precursors for optoelectronic materials .
Data contradiction : Cu catalysts underperform compared to Pd systems due to slower oxidative addition kinetics .
Q. Advanced: How do computational methods aid in predicting reactivity trends for this compound?
- DFT calculations (e.g., Gaussian 16) model transition states for cross-coupling reactions. The electron-withdrawing ester group lowers the LUMO energy, favoring nucleophilic attack at C4.
- Molecular docking (AutoDock Vina) predicts binding affinities for drug-discovery analogs. For example, thiophene derivatives show potential as kinase inhibitors .
Validation : Compare computed HOMO-LUMO gaps with experimental cyclic voltammetry data.
Q. Advanced: How to resolve contradictions in crystallographic data for this compound derivatives?
- Issue : Disordered solvent molecules or twinning can distort refinement.
- Solution : Use SHELXL’s TWIN/BASF commands to model twinning and PART/ISOR to handle disorder .
- Case study : Ethyl 4-(3-bromo-2-thienyl) derivatives required anisotropic displacement parameter (ADP) constraints to refine Br···S interactions .
Q. Basic: What are the best practices for synthesizing this compound with >95% purity?
- Step 1 : Brominate thiophene-3-carboxylate using NBS (N-bromosuccinimide) in CCl₄ under UV light.
- Step 2 : Purify via column chromatography (silica gel, hexane/EtOAc 4:1) and confirm purity by GC-MS or HPLC .
Pitfall : Residual solvents (e.g., DMF) can coordinate metal catalysts, reducing reaction yields.
Q. Advanced: How to design experiments analyzing the photophysical properties of this compound derivatives?
Comparison with Similar Compounds
Ethyl 4-bromothiophene-3-carboxylate belongs to a broader class of brominated heterocyclic carboxylates. Below, it is compared structurally and functionally with analogs differing in substituent positions, heterocyclic cores, and functional groups.
Substituent Position Variants
- The 4-bromo substitution in the target compound likely enhances electrophilic aromatic substitution (EAS) reactivity at the 5-position due to the electron-withdrawing ester group at C3 .
- Ethyl 4-bromo-2-carboxylate derivatives : Thiophene-2-carboxylates (e.g., butyl 4-bromothiophene-3-carboxylate in ) exhibit distinct regioselectivity in reactions. For example, Cu-catalyzed coupling with ethyl 2-mercaptoacetate failed for thiophene-3-carboxylates but succeeded for 2-carboxylate analogs .
Ester Group Variants
- Pd-catalyzed systems, however, improved coupling efficiency, highlighting the role of catalyst selection in overcoming steric effects .
Heterocyclic Core Variants
Functional Group Variants
- Ethyl 4-bromomethylthiophene-3-carboxylate : Bromine as a methyl substituent (e.g., in ’s HXO-43984) enables alkylation reactions, contrasting with the aryl bromide’s utility in cross-couplings .
- Ethyl 4-(4-chlorophenyl)-2-[(cyanoacetyl)amino]thiophene-3-carboxylate (): Chlorine substituents reduce leaving-group ability compared to bromine, making the target compound more reactive in nucleophilic substitutions .
Key Research Findings
- Catalytic Reactivity: Pd-catalyzed systems outperform Cu-based methods for coupling this compound derivatives, as demonstrated in the synthesis of thieno[3,4-b]thiophene frameworks .
- Electronic Effects : The electron-withdrawing ester group at C3 directs electrophilic substitution to C5 in the thiophene ring, a feature absent in benzene or pyridine analogs .
- Steric Influence : Ethyl esters exhibit superior reactivity to bulkier esters (e.g., butyl) in cross-couplings due to reduced steric hindrance .
Preparation Methods
Direct Bromination of Ethyl Thiophene-3-carboxylate
The most straightforward approach involves electrophilic bromination of ethyl thiophene-3-carboxylate. The ester group at position 3 directs electrophilic substitution to the 4-position due to its meta-directing nature.
Brominating Agents and Conditions
N-Bromosuccinimide (NBS) in polar aprotic solvents like dimethylformamide (DMF) or dichloromethane (DCM) is widely employed. For example, reacting ethyl thiophene-3-carboxylate with 1.1 equivalents of NBS at 0–5°C for 6–8 hours yields 72–85% of the target compound . Alternatively, bromine (Br₂) in acetic acid at 40°C achieves comparable yields but requires careful handling due to bromine’s volatility and toxicity .
Table 1: Comparative Bromination Methods
Brominating Agent | Solvent | Temperature (°C) | Time (h) | Yield (%) |
---|---|---|---|---|
NBS | DMF | 0–5 | 6–8 | 85 |
Br₂ | Acetic Acid | 40 | 4 | 78 |
HBr/H₂O₂ | DCM | 25 | 12 | 65 |
Mechanistic Considerations
The reaction proceeds via generation of a bromonium ion intermediate, stabilized by the electron-withdrawing ester group. Steric effects from the ethyl ester minimize dibromination, though excess bromine or prolonged reaction times can lead to 2,4-dibromo byproducts. Purification via silica gel chromatography (hexane/ethyl acetate, 4:1) typically isolates the monobrominated product in >95% purity .
Ring-Synthesis Approaches
Constructing the thiophene core with pre-installed bromine and ester groups offers an alternative to direct bromination.
Gewald Reaction Modifications
The classic Gewald reaction, which condenses ketones, cyanoacetates, and sulfur, can be adapted. Using 4-bromo-3-oxobutanoic acid ethyl ester and elemental sulfur in ethanol under reflux forms the thiophene ring in one pot. This method achieves 60–70% yield but requires stringent moisture control to prevent hydrolysis of the ester.
Palladium-Catalyzed Cross-Coupling
While less common, Suzuki-Miyaura coupling has been explored for functionalizing thiophene precursors. For instance, ethyl 3-bromothiophene-4-carboxylate undergoes coupling with aryl boronic acids, but this route is more relevant to derivatives rather than the parent compound .
Esterification of 4-Bromothiophene-3-carboxylic Acid
For laboratories with access to 4-bromothiophene-3-carboxylic acid, esterification via Fischer–Speier conditions (H₂SO₄, ethanol, reflux) provides high yields (>90%). However, the carboxylic acid precursor is often less accessible, making this method niche .
Analytical Characterization
Key spectroscopic data for this compound:
Properties
IUPAC Name |
ethyl 4-bromothiophene-3-carboxylate | |
---|---|---|
Details | Computed by LexiChem 2.6.6 (PubChem release 2019.06.18) | |
Source | PubChem | |
URL | https://pubchem.ncbi.nlm.nih.gov | |
Description | Data deposited in or computed by PubChem | |
InChI |
InChI=1S/C7H7BrO2S/c1-2-10-7(9)5-3-11-4-6(5)8/h3-4H,2H2,1H3 | |
Details | Computed by InChI 1.0.5 (PubChem release 2019.06.18) | |
Source | PubChem | |
URL | https://pubchem.ncbi.nlm.nih.gov | |
Description | Data deposited in or computed by PubChem | |
InChI Key |
LARLHLAYBCZHLV-UHFFFAOYSA-N | |
Details | Computed by InChI 1.0.5 (PubChem release 2019.06.18) | |
Source | PubChem | |
URL | https://pubchem.ncbi.nlm.nih.gov | |
Description | Data deposited in or computed by PubChem | |
Canonical SMILES |
CCOC(=O)C1=CSC=C1Br | |
Details | Computed by OEChem 2.1.5 (PubChem release 2019.06.18) | |
Source | PubChem | |
URL | https://pubchem.ncbi.nlm.nih.gov | |
Description | Data deposited in or computed by PubChem | |
Molecular Formula |
C7H7BrO2S | |
Details | Computed by PubChem 2.1 (PubChem release 2019.06.18) | |
Source | PubChem | |
URL | https://pubchem.ncbi.nlm.nih.gov | |
Description | Data deposited in or computed by PubChem | |
Molecular Weight |
235.10 g/mol | |
Details | Computed by PubChem 2.1 (PubChem release 2021.05.07) | |
Source | PubChem | |
URL | https://pubchem.ncbi.nlm.nih.gov | |
Description | Data deposited in or computed by PubChem | |
Synthesis routes and methods I
Procedure details
Synthesis routes and methods II
Procedure details
Retrosynthesis Analysis
AI-Powered Synthesis Planning: Our tool employs the Template_relevance Pistachio, Template_relevance Bkms_metabolic, Template_relevance Pistachio_ringbreaker, Template_relevance Reaxys, Template_relevance Reaxys_biocatalysis model, leveraging a vast database of chemical reactions to predict feasible synthetic routes.
One-Step Synthesis Focus: Specifically designed for one-step synthesis, it provides concise and direct routes for your target compounds, streamlining the synthesis process.
Accurate Predictions: Utilizing the extensive PISTACHIO, BKMS_METABOLIC, PISTACHIO_RINGBREAKER, REAXYS, REAXYS_BIOCATALYSIS database, our tool offers high-accuracy predictions, reflecting the latest in chemical research and data.
Strategy Settings
Precursor scoring | Relevance Heuristic |
---|---|
Min. plausibility | 0.01 |
Model | Template_relevance |
Template Set | Pistachio/Bkms_metabolic/Pistachio_ringbreaker/Reaxys/Reaxys_biocatalysis |
Top-N result to add to graph | 6 |
Feasible Synthetic Routes
Disclaimer and Information on In-Vitro Research Products
Please be aware that all articles and product information presented on BenchChem are intended solely for informational purposes. The products available for purchase on BenchChem are specifically designed for in-vitro studies, which are conducted outside of living organisms. In-vitro studies, derived from the Latin term "in glass," involve experiments performed in controlled laboratory settings using cells or tissues. It is important to note that these products are not categorized as medicines or drugs, and they have not received approval from the FDA for the prevention, treatment, or cure of any medical condition, ailment, or disease. We must emphasize that any form of bodily introduction of these products into humans or animals is strictly prohibited by law. It is essential to adhere to these guidelines to ensure compliance with legal and ethical standards in research and experimentation.