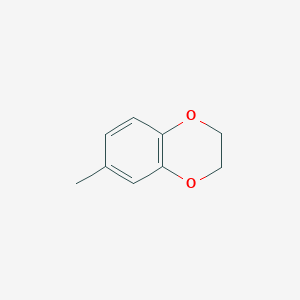
6-Methyl-2,3-dihydro-1,4-benzodioxine
Overview
Description
“6-Methyl-2,3-dihydro-1,4-benzodioxine” is a chemical compound with the molecular formula C9H10O2 . It is a heterocyclic compound containing a benzene ring fused to a 1,4-dioxane ring .
Synthesis Analysis
The synthesis of “this compound” involves several steps. The upstream raw materials include 1,2-Dibromoethane and 4-methylcatechol . The exact process and conditions for the synthesis may vary and are often proprietary to the manufacturer .Molecular Structure Analysis
The molecular structure of “this compound” consists of nine carbon atoms, ten hydrogen atoms, and two oxygen atoms . The exact structure can be determined using various spectroscopic techniques.Chemical Reactions Analysis
The chemical reactions involving “this compound” are complex and depend on the conditions and reagents used. For example, it can react with bromomethyl to form 2-(Bromomethyl)-6-methyl-2,3-dihydro-1,4-benzodioxine .Physical And Chemical Properties Analysis
The physical and chemical properties of “this compound” include a molecular weight of 150.17400 . The exact density, boiling point, and melting point are not available .properties
IUPAC Name |
6-methyl-2,3-dihydro-1,4-benzodioxine | |
---|---|---|
Details | Computed by LexiChem 2.6.6 (PubChem release 2019.06.18) | |
Source | PubChem | |
URL | https://pubchem.ncbi.nlm.nih.gov | |
Description | Data deposited in or computed by PubChem | |
InChI |
InChI=1S/C9H10O2/c1-7-2-3-8-9(6-7)11-5-4-10-8/h2-3,6H,4-5H2,1H3 | |
Details | Computed by InChI 1.0.5 (PubChem release 2019.06.18) | |
Source | PubChem | |
URL | https://pubchem.ncbi.nlm.nih.gov | |
Description | Data deposited in or computed by PubChem | |
InChI Key |
AYTGARGOCPEHGL-UHFFFAOYSA-N | |
Details | Computed by InChI 1.0.5 (PubChem release 2019.06.18) | |
Source | PubChem | |
URL | https://pubchem.ncbi.nlm.nih.gov | |
Description | Data deposited in or computed by PubChem | |
Canonical SMILES |
CC1=CC2=C(C=C1)OCCO2 | |
Details | Computed by OEChem 2.1.5 (PubChem release 2019.06.18) | |
Source | PubChem | |
URL | https://pubchem.ncbi.nlm.nih.gov | |
Description | Data deposited in or computed by PubChem | |
Molecular Formula |
C9H10O2 | |
Details | Computed by PubChem 2.1 (PubChem release 2019.06.18) | |
Source | PubChem | |
URL | https://pubchem.ncbi.nlm.nih.gov | |
Description | Data deposited in or computed by PubChem | |
DSSTOX Substance ID |
DTXSID30496452 | |
Record name | 6-Methyl-2,3-dihydro-1,4-benzodioxine | |
Source | EPA DSSTox | |
URL | https://comptox.epa.gov/dashboard/DTXSID30496452 | |
Description | DSSTox provides a high quality public chemistry resource for supporting improved predictive toxicology. | |
Molecular Weight |
150.17 g/mol | |
Details | Computed by PubChem 2.1 (PubChem release 2021.05.07) | |
Source | PubChem | |
URL | https://pubchem.ncbi.nlm.nih.gov | |
Description | Data deposited in or computed by PubChem | |
CAS RN |
33632-35-8 | |
Record name | 2,3-Dihydro-6-methyl-1,4-benzodioxin | |
Source | CAS Common Chemistry | |
URL | https://commonchemistry.cas.org/detail?cas_rn=33632-35-8 | |
Description | CAS Common Chemistry is an open community resource for accessing chemical information. Nearly 500,000 chemical substances from CAS REGISTRY cover areas of community interest, including common and frequently regulated chemicals, and those relevant to high school and undergraduate chemistry classes. This chemical information, curated by our expert scientists, is provided in alignment with our mission as a division of the American Chemical Society. | |
Explanation | The data from CAS Common Chemistry is provided under a CC-BY-NC 4.0 license, unless otherwise stated. | |
Record name | 6-Methyl-2,3-dihydro-1,4-benzodioxine | |
Source | EPA DSSTox | |
URL | https://comptox.epa.gov/dashboard/DTXSID30496452 | |
Description | DSSTox provides a high quality public chemistry resource for supporting improved predictive toxicology. | |
Synthesis routes and methods I
Procedure details
Synthesis routes and methods II
Procedure details
Disclaimer and Information on In-Vitro Research Products
Please be aware that all articles and product information presented on BenchChem are intended solely for informational purposes. The products available for purchase on BenchChem are specifically designed for in-vitro studies, which are conducted outside of living organisms. In-vitro studies, derived from the Latin term "in glass," involve experiments performed in controlled laboratory settings using cells or tissues. It is important to note that these products are not categorized as medicines or drugs, and they have not received approval from the FDA for the prevention, treatment, or cure of any medical condition, ailment, or disease. We must emphasize that any form of bodily introduction of these products into humans or animals is strictly prohibited by law. It is essential to adhere to these guidelines to ensure compliance with legal and ethical standards in research and experimentation.