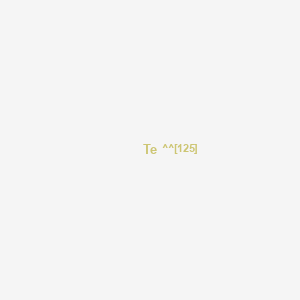
Tellurium-125
Overview
Description
Tellurium-125 (¹²⁵Te) is a stable, non-radioactive isotope of tellurium. It is notable for its role in nuclear chemistry as the decay product of iodine-125 (¹²⁵I), a radioisotope used in medical applications such as cancer therapy . ¹²⁵I decays via electron capture with a half-life of 59.4 days, emitting low-energy electrons (35.5 keV) and ultimately forming ¹²⁵Te . Unlike its radioactive precursor, ¹²⁵Te is chemically stable, making it valuable in spectroscopic studies (e.g., NMR, Mössbauer) to elucidate structural and electronic properties of tellurium-containing compounds .
Preparation Methods
Synthetic Routes and Reaction Conditions: Tellurium-125 can be synthesized through various methods, including the reduction of tellurium dioxide with hydrogen or carbon at high temperatures. Another method involves the electrochemical reduction of tellurium compounds in an acidic medium .
Industrial Production Methods: In industrial settings, tellurium is often obtained as a byproduct of copper refining. The process involves the extraction of tellurium from copper anode slimes, followed by purification through methods such as zone refining and hydrometallurgical techniques . Zone refining is particularly effective in achieving high-purity tellurium by removing impurities through repeated melting and solidification cycles .
Chemical Reactions Analysis
Types of Reactions: Tellurium-125 undergoes various chemical reactions, including oxidation, reduction, and substitution reactions.
Common Reagents and Conditions:
Oxidation: this compound can be oxidized using strong oxidizing agents such as nitric acid or hydrogen peroxide, forming tellurium dioxide.
Reduction: Reduction of tellurium compounds can be achieved using reducing agents like hydrogen or carbon at elevated temperatures.
Substitution: this compound can participate in substitution reactions with halogens, forming tellurium halides.
Major Products:
Oxidation: Tellurium dioxide (TeO2)
Reduction: Elemental tellurium (Te)
Substitution: Tellurium halides (e.g., tellurium tetrachloride, TeCl4)
Scientific Research Applications
Nuclear Magnetic Resonance (NMR) Spectroscopy
Chemical Shift Analysis
One of the primary applications of Te is in NMR spectroscopy. The isotope exhibits a large chemical shift window of approximately 5000 ppm, making it highly sensitive to the electronic environment surrounding the tellurium nucleus. This sensitivity allows researchers to investigate the electronic structures of organotellurium compounds effectively. Studies have shown that the chemical shifts can be correlated with structural motifs, providing insights into molecular interactions and bonding characteristics .
Case Study: Organotellurium Compounds
Research has demonstrated that Te NMR can be used to analyze a series of organotellurium compounds, revealing how substituents affect chemical shielding. The analysis indicated that the deshielding effect is influenced by the electronic properties of substituents and their interactions with tellurium’s lone pairs . This application is crucial for understanding the reactivity and stability of these compounds in various chemical environments.
Mössbauer Spectroscopy
Investigation of Oxides
Te is also utilized in Mössbauer spectroscopy to study tellurium oxides and their mixed compounds. The Mössbauer spectra provide valuable information about the oxidation states and local environments of tellurium within these materials. For instance, studies on tellurium(IV)-oxygen compounds have highlighted variations in electric quadrupole interactions, which are essential for understanding the bonding characteristics in these systems .
Case Study: Telluride Compounds
In a detailed investigation involving bis(trifluoromethyl)- and bis(pentafluorophenyl)-tellurides, Te Mössbauer spectroscopy revealed insights into bonding effects and molecular dynamics. The results indicated minimal transmission of bonding effects through the tin-tellurium bond, which has implications for designing materials with specific electronic properties .
Biomedical Applications
Radiolabeling for Imaging
Although Te itself is stable, it can be used as a radiolabel for biological and biomedical applications. Its isotopic properties enable it to serve as a tracer in imaging studies, particularly in positron emission tomography (PET). This application is critical for tracking biological processes and understanding disease mechanisms at a molecular level .
Case Study: Drug Development
Recent studies have explored methods for producing metastable Te as part of drug development pathways. These methods could enhance the efficacy of drug formulations by improving their imaging capabilities during clinical trials .
Material Science
Thin Film Applications
In material science, Te is employed in producing thin films and nanoparticles for various applications, including electronics and photonics. Its unique electrical properties make it suitable for use in semiconductor devices and photovoltaic cells .
Case Study: Nanoparticle Synthesis
Research has indicated that Te nanoparticles can be synthesized with controlled sizes and morphologies, which are essential for optimizing their performance in electronic applications. Such advancements open pathways for developing next-generation materials with enhanced functionalities .
Summary Table of Applications
Mechanism of Action
Tellurium-125 is often compared with other chalcogen elements such as sulfur and selenium due to their similar chemical properties. this compound exhibits unique characteristics that distinguish it from its counterparts:
Sulfur:
- Sulfur is more abundant and less toxic compared to tellurium.
- This compound has a higher atomic weight and different electronic properties, making it suitable for specific applications in electronics and materials science .
Selenium:
- Selenium shares similar chemical properties with tellurium but has different biological roles and toxicity profiles.
- This compound is preferred in certain industrial applications due to its superior thermoelectric properties .
Comparison with Similar Compounds
Organotellurium Compounds: Oxidation States and NMR Sensitivity
¹²⁵Te nuclear magnetic resonance (NMR) is a critical tool for studying organotellurium compounds. Key findings include:
- Te(II) vs. Te(IV) Oxidation States: In tellurapyrans, tellurapyranones, and tellurapyrylium salts, ¹²⁵Te NMR chemical shifts are highly sensitive to oxidation states. For example, Te(II) compounds exhibit significant shifts in the presence of a formal positive charge, while Te(IV) complexes show distinct shifts due to differences in electron density .
- Te-P Coupling Constants : In phosphine tellurides and tellurophosphines, ¹²⁵Te NMR reveals strong coupling constants (e.g., Te-P couplings up to 1,500 Hz), reflecting robust bonding interactions between tellurium and phosphorus .
Table 1: ¹²⁵Te NMR Characteristics in Selected Organotellurium Compounds
Compound Class | Oxidation State | Key NMR Feature | Reference |
---|---|---|---|
Tellurapyrylium salts | Te(II) | Shifts sensitive to positive charge | |
Phosphine tellurides | Te(0)/Te(II) | High Te-P coupling constants (~1,500 Hz) |
Inorganic Tellurium Complexes: Mössbauer Spectroscopy Insights
Mössbauer spectroscopy of ¹²⁵Te-enriched complexes highlights trends in isomer shifts:
- Halide Complexes : For TeCl₆²⁻, TeBr₆²⁻, and TeI₆²⁻, isomer shifts are determined primarily by the anion’s electronic structure, with minimal influence from cations. For instance, TeCl₆²⁻ exhibits a distinct isomer shift compared to TeBr₆²⁻, reflecting differences in ligand electronegativity .
- Fluoride Complexes : The TeF₅⁻ anion (in CsTeF₅) displays a Mössbauer signature distinct from TeF₆²⁻, confirming its unique geometry and bonding .
Table 2: Mössbauer Isomer Shifts in Inorganic Tellurium Complexes
Complex | Isomer Shift Trend | Key Observation | Reference |
---|---|---|---|
TeCl₆²⁻ | Higher than TeBr₆²⁻ | Ligand electronegativity effect | |
CsTeF₅ | Distinct from TeF₆²⁻ | Confirms TeF₅⁻ geometry |
Comparison with Selenium Analogs
While selenium (Se) shares chemical similarities with tellurium, key differences arise:
- Literature and Synthesis : Tellurium compounds, including ¹²⁵Te derivatives, are less studied than selenium analogs due to challenges in synthesis and stability .
- Spectroscopic Sensitivity : ¹²⁵Te NMR exhibits larger chemical shift ranges (~3,000 ppm) compared to ⁷⁷Se NMR (~1,000 ppm), enabling finer resolution of electronic environments .
Radioactive vs. Non-Radioactive Tellurium: ¹²⁵Te vs. ¹²⁵I
¹²⁵Te and ¹²⁵I form a complementary pair in nuclear chemistry:
- Decay Relationship : ¹²⁵I decays to ¹²⁵Te via electron capture, releasing therapeutic low-energy electrons .
- Applications : ¹²⁵I is used in brachytherapy, while ¹²⁵Te serves as a stable marker in structural studies .
Table 3: Comparative Properties of ¹²⁵Te and ¹²⁵I
Property | ¹²⁵Te | ¹²⁵I |
---|---|---|
Radioactivity | Non-radioactive | Radioactive (t₁/₂ = 59.4 days) |
Primary Use | Spectroscopic studies | Cancer therapy |
Decay Mode | Stable | Electron capture → ¹²⁵Te |
Key Energy | N/A | 35.5 keV electrons |
Reference |
Biological Activity
Tellurium-125 () is a stable isotope of tellurium, which has garnered interest in various fields, including biological and biomedical research. This article explores the biological activity associated with , focusing on its chemical properties, biological interactions, and potential applications based on recent studies.
Tellurium is a member of the chalcogen group, and its isotopes, particularly , are studied using techniques like nuclear magnetic resonance (NMR) spectroscopy. The sensitivity of NMR allows for detailed investigations into the structure and dynamics of organotellurium compounds.
Key NMR Characteristics of
Property | Value |
---|---|
Spin | 1/2 |
Natural abundance | 7.07% |
Chemical shift range | 5800 ppm (from -1400 to 3400) |
Receptivity relative to (natural abundance) | |
Receptivity relative to (natural abundance) | 0.961 |
The chemical shifts observed in are influenced by the electronic environment surrounding the tellurium atom, making it a valuable probe for studying molecular interactions in biological systems .
Biological Interactions and Toxicity
While tellurium itself does not have a known biological function, its compounds exhibit significant biological activity. Recent studies indicate that tellurium oxyanions can exert toxic effects on both prokaryotic and eukaryotic organisms. The toxicity mechanisms often involve the disruption of essential biological processes such as ATP synthesis and protein function.
Mechanisms of Toxicity
- Inhibition of ATP Synthesis : Tellurite () disrupts transmembrane proton gradients in bacterial cells, leading to decreased ATP production .
- Protein Synthesis Disruption : Tellurium compounds can interfere with proteins that contain thiol groups, leading to altered protein activity or inactivity .
- Oxidative Stress : The presence of tellurium compounds can increase reactive oxygen species (ROS), causing further cellular damage .
Resistance Mechanisms in Microorganisms
Certain microorganisms have developed resistance mechanisms against tellurium toxicity. These include:
- Reduction of Tellurite : Some bacteria can reduce toxic tellurite to less harmful elemental tellurium, effectively detoxifying their environment .
- Gene Clusters for Resistance : Genetic determinants conferring resistance have been identified in various bacterial strains, showcasing a diverse array of resistance strategies .
Case Study 1: Tellurite Effects on E. coli
A study demonstrated that E. coli strains exposed to tellurite showed inhibited growth and ATP depletion. However, certain resistant strains exhibited increased protein synthesis in response to tellurite exposure, suggesting a complex interaction between toxicity and microbial adaptation .
Case Study 2: Tellurium Compounds in Cancer Research
Research has indicated that tellurium compounds may play a role in cancer therapy due to their ability to induce oxidative stress selectively in cancer cells while sparing normal cells. This selectivity is attributed to differential uptake and metabolism of tellurium compounds by cancerous tissues .
Applications in Biomedical Research
The unique properties of make it suitable for various applications in biomedical research:
Q & A
Basic Research Questions
Q. What are the primary methods for synthesizing Tellurium-125 in laboratory settings?
this compound is primarily synthesized via the radioactive decay of Iodine-125 through electron capture, where a proton and electron combine to form a neutron. This decay process can be observed using advanced techniques such as scanning tunneling microscopy (STM), which enables atomic-level visualization of individual iodine atoms transitioning into tellurium . Researchers should prepare samples by depositing iodine-125 on gold substrates in controlled environments to minimize contamination and facilitate bonding during evaporation .
Q. What spectroscopic techniques are most effective for characterizing this compound’s electronic structure?
X-ray photoelectron spectroscopy (XPS) and transmission electron microscopy (TEM) are widely used to analyze the electronic configuration and isotopic purity of this compound. For quantitative isotopic analysis, inductively coupled plasma mass spectrometry (ICP-MS) provides high sensitivity. Researchers must calibrate instruments using certified reference materials and account for potential interferences from other tellurium isotopes .
Q. What safety protocols are essential when handling this compound in laboratory environments?
Due to its acute toxicity and potential for skin sensitization, laboratories must adhere to strict safety measures:
- Use fume hoods and glove boxes to prevent inhalation or dermal exposure.
- Employ personal protective equipment (PPE), including nitrile gloves and lab coats.
- Store samples in sealed, labeled containers under inert atmospheres to avoid oxidation .
Advanced Research Questions
Q. How can researchers address discrepancies in measured half-lives of this compound across experimental setups?
Variations in reported half-lives may arise from differences in detection sensitivity, environmental conditions (e.g., temperature, humidity), or isotopic impurities. To mitigate these:
- Standardize calibration protocols using traceable reference materials.
- Perform replicate experiments with statistical analysis (e.g., Poisson distribution for decay event modeling).
- Validate results through peer-reviewed replication studies .
Q. What are the challenges in isolating this compound from other tellurium isotopes, and what separation techniques are recommended?
Isotopic separation is complicated by the similar physical properties of tellurium isotopes. Effective methods include:
- Gas centrifugation : Leverages mass differences for partial enrichment.
- Laser ionization : Selectively excites this compound atoms for separation.
- Chemical exchange : Utilizes isotopic fractionation in redox reactions. Post-separation, purity must be verified via high-resolution mass spectrometry .
Q. How does the stochastic nature of this compound’s decay events impact single-atom observation experiments?
The random decay of individual atoms requires long-term tracking and robust data acquisition systems. Researchers should:
- Use time-lapsed STM imaging to capture decay events over weeks.
- Apply Monte Carlo simulations to model decay probabilities and validate observational data.
- Control environmental variables (e.g., vacuum stability) to reduce noise .
Q. What experimental designs optimize the study of this compound’s chemical reactivity in organometallic complexes?
To investigate reactivity:
- Synthesize this compound complexes with ligands (e.g., thiols, amines) under anaerobic conditions.
- Monitor reaction kinetics using nuclear magnetic resonance (NMR) with isotopic labeling.
- Compare reactivity trends with stable tellurium isotopes to identify mass-dependent effects .
Q. Methodological Considerations for Data Analysis
Q. What statistical approaches are recommended for analyzing decay kinetics in this compound?
- Bayesian inference : Quantifies uncertainty in decay rate constants.
- Maximum likelihood estimation (MLE) : Fits decay curves to experimental data.
- Chi-squared tests : Assesses goodness-of-fit between observed and theoretical models .
Q. How should researchers design experiments to minimize isotopic cross-contamination during this compound synthesis?
Properties
IUPAC Name |
tellurium-125 | |
---|---|---|
Source | PubChem | |
URL | https://pubchem.ncbi.nlm.nih.gov | |
Description | Data deposited in or computed by PubChem | |
InChI |
InChI=1S/Te/i1-3 | |
Source | PubChem | |
URL | https://pubchem.ncbi.nlm.nih.gov | |
Description | Data deposited in or computed by PubChem | |
InChI Key |
PORWMNRCUJJQNO-OIOBTWANSA-N | |
Source | PubChem | |
URL | https://pubchem.ncbi.nlm.nih.gov | |
Description | Data deposited in or computed by PubChem | |
Canonical SMILES |
[Te] | |
Source | PubChem | |
URL | https://pubchem.ncbi.nlm.nih.gov | |
Description | Data deposited in or computed by PubChem | |
Isomeric SMILES |
[125Te] | |
Source | PubChem | |
URL | https://pubchem.ncbi.nlm.nih.gov | |
Description | Data deposited in or computed by PubChem | |
Molecular Formula |
Te | |
Source | PubChem | |
URL | https://pubchem.ncbi.nlm.nih.gov | |
Description | Data deposited in or computed by PubChem | |
Molecular Weight |
124.90443 g/mol | |
Source | PubChem | |
URL | https://pubchem.ncbi.nlm.nih.gov | |
Description | Data deposited in or computed by PubChem | |
CAS No. |
14390-73-9 | |
Record name | Tellurium, isotope of mass 125 | |
Source | ChemIDplus | |
URL | https://pubchem.ncbi.nlm.nih.gov/substance/?source=chemidplus&sourceid=0014390739 | |
Description | ChemIDplus is a free, web search system that provides access to the structure and nomenclature authority files used for the identification of chemical substances cited in National Library of Medicine (NLM) databases, including the TOXNET system. | |
Disclaimer and Information on In-Vitro Research Products
Please be aware that all articles and product information presented on BenchChem are intended solely for informational purposes. The products available for purchase on BenchChem are specifically designed for in-vitro studies, which are conducted outside of living organisms. In-vitro studies, derived from the Latin term "in glass," involve experiments performed in controlled laboratory settings using cells or tissues. It is important to note that these products are not categorized as medicines or drugs, and they have not received approval from the FDA for the prevention, treatment, or cure of any medical condition, ailment, or disease. We must emphasize that any form of bodily introduction of these products into humans or animals is strictly prohibited by law. It is essential to adhere to these guidelines to ensure compliance with legal and ethical standards in research and experimentation.