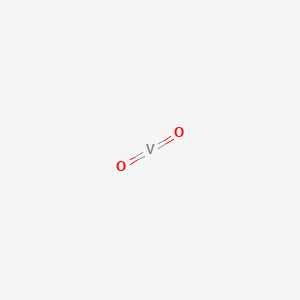
Vanadium dioxide
Overview
Description
Vanadium dioxide, also known as vanadium(IV) oxide, is an inorganic compound with the chemical formula VO₂. It is a dark blue solid that exhibits unique properties, such as a metal-insulator transition at approximately 68°C (341 K). This transition is characterized by a dramatic change in electrical conductivity and optical properties, making this compound a material of significant interest in various scientific and industrial applications .
Mechanism of Action
Target of Action
Vanadium dioxide (VO2) primarily targets thermochromic smart window materials . It has been widely investigated due to its unique properties, such as its ability to modulate solar transmission . It also targets thin films and nanostructures and has been used in surface coating, sensors, and imaging .
Mode of Action
VO2 exhibits a metal-insulator transition (MIT) . This transition is characterized by a change from a room-temperature monoclinic insulating phase to a high-temperature rutile metallic phase . The phase transition of VO2 is accompanied by sudden changes in conductance and optical transmittance . This unique property allows VO2 to be used in a variety of applications, including memory devices, phase-change switches, passive radiative cooling applications, aerospace communication systems, and neuromorphic computing .
Biochemical Pathways
While VO2 is primarily used in materials science, vanadium compounds have been shown to have biochemical effects. For instance, vanadium acts as an insulin mimetic and enhances glucose and lipid metabolism when administered at lower doses . It inhibits the intracellular enzyme protein tyrosine phosphatase, facilitating the uptake of glucose inside the cell .
Pharmacokinetics
It’s known that the properties of vo2 can be influenced by doping with other elements . For example, doping VO2 with tungsten (W) reduces the transition temperature, while doping with gallium (Ga) can increase it .
Result of Action
The primary result of VO2’s action is its phase transition . At temperatures below 67°C, VO2 has a monoclinic crystal structure. When heated to more than 67°C, it transitions to a tetragonal structure . At the same temperature, crystalline VO2 converts from an electrical insulator to a conductor . This property has been used to create new field-effect transistors .
Action Environment
The action of VO2 is highly dependent on environmental factors. For instance, the phase transition temperature can be influenced by external stimuli such as heat or stress . Moreover, the properties of VO2 can be modulated by the physiological environment, such as pH and redox conditions . In water, vanadium forms either aqua-complexes or oxo-cations with typical colors .
Biochemical Analysis
Biochemical Properties
Vanadium dioxide interacts with various biomolecules, leading to changes in biochemical reactions . For instance, it has been shown to interact with enzymes and proteins, influencing their function and activity . In particular, vanadium compounds have been found to inhibit protein tyrosine phosphatases, which play a crucial role in cellular signaling pathways .
Cellular Effects
This compound has significant effects on various types of cells and cellular processes . For example, it has been found to inhibit cell proliferation and induce apoptosis in certain cell lines . It can also cause endoplasmic reticulum stress, leading to the release of calcium ions and the overproduction of reactive oxygen species . These effects can result in DNA damage, mitochondrial damage, autophagy, and lysosomal damage, ultimately leading to apoptosis and inhibition of cell proliferation .
Molecular Mechanism
The molecular mechanism of action of this compound involves several key processes . At the molecular level, it exerts its effects through binding interactions with biomolecules and changes in gene expression . For instance, it can inhibit the activity of certain enzymes, leading to changes in cellular signaling pathways .
Temporal Effects in Laboratory Settings
In laboratory settings, the effects of this compound can change over time . For instance, it has been observed that the compound’s stability, degradation, and long-term effects on cellular function can vary in in vitro or in vivo studies .
Dosage Effects in Animal Models
The effects of this compound can vary with different dosages in animal models . For instance, it has been observed that higher dosages lead to higher vanadium contents in organs . Moreover, toxic or adverse effects have been reported at high doses .
Metabolic Pathways
This compound is involved in various metabolic pathways . It interacts with enzymes and cofactors, and can have effects on metabolic flux or metabolite levels .
Transport and Distribution
This compound is transported and distributed within cells and tissues . It can interact with transporters or binding proteins, and can have effects on its localization or accumulation .
Preparation Methods
Synthetic Routes and Reaction Conditions: Vanadium dioxide can be synthesized through several methods, including:
Chemical Vapor Deposition (CVD): This method involves the reaction of vanadium precursors with oxygen at high temperatures to form this compound thin films.
Physical Vapor Deposition (PVD): In this technique, vanadium is vaporized and then deposited onto a substrate, where it reacts with oxygen to form this compound.
Sol-Gel Method: This involves the hydrolysis and condensation of vanadium alkoxides to form a gel, which is then calcined to produce this compound.
Industrial Production Methods: Industrial production of this compound typically employs the CVD method due to its ability to produce high-purity thin films with controlled properties. The reaction conditions include temperatures ranging from 500°C to 700°C and the use of vanadium tetrachloride as a precursor .
Chemical Reactions Analysis
Types of Reactions: Vanadium dioxide undergoes various chemical reactions, including:
Reduction: It can be reduced to vanadium(III) oxide (V₂O₃) using reducing agents like hydrogen gas.
Amphoteric Reactions: this compound is amphoteric, dissolving in non-oxidizing acids to form the blue vanadyl ion ([VO]²⁺) and in alkali to form the brown [V₄O₉]²⁻ ion.
Common Reagents and Conditions:
Oxidation: Potassium permanganate in acidic conditions.
Reduction: Hydrogen gas at elevated temperatures.
Amphoteric Reactions: Non-oxidizing acids (e.g., hydrochloric acid) and alkali (e.g., sodium hydroxide).
Major Products:
Oxidation: Vanadium pentoxide (V₂O₅).
Reduction: Vanadium(III) oxide (V₂O₃).
Amphoteric Reactions: Vanadyl ion ([VO]²⁺) and [V₄O₉]²⁻ ion.
Scientific Research Applications
Vanadium dioxide has a wide range of scientific research applications, including:
Smart Windows: Due to its thermochromic properties, this compound is used in smart windows that can regulate heat and light transmission based on temperature.
Sensors: Its phase transition properties make it suitable for use in temperature and pressure sensors.
Memory Devices: this compound is used in non-volatile memory devices due to its ability to switch between insulating and metallic states.
Neuromorphic Computing: It is explored for use in neuromorphic computing systems that mimic the human brain’s neural networks.
Aerospace Communication Systems: this compound is used in programmable radiofrequency electronic functions for aerospace communication systems.
Comparison with Similar Compounds
Vanadium dioxide is part of a family of vanadium oxides, each with distinct properties:
Vanadium Monoxide (VO): Exhibits metallic properties at all temperatures.
Vanadium Sesquioxide (V₂O₃): Undergoes a metal-insulator transition at lower temperatures compared to this compound.
Vanadium Pentoxide (V₂O₅): Does not exhibit a metal-insulator transition but is known for its catalytic properties.
Uniqueness of this compound: this compound’s near-room-temperature metal-insulator transition and its dramatic changes in electrical and optical properties make it unique among vanadium oxides. This property is not observed in vanadium monoxide or vanadium pentoxide, making this compound particularly valuable for applications requiring temperature-sensitive switching .
Properties
IUPAC Name |
dioxovanadium | |
---|---|---|
Source | PubChem | |
URL | https://pubchem.ncbi.nlm.nih.gov | |
Description | Data deposited in or computed by PubChem | |
InChI |
InChI=1S/2O.V | |
Source | PubChem | |
URL | https://pubchem.ncbi.nlm.nih.gov | |
Description | Data deposited in or computed by PubChem | |
InChI Key |
GRUMUEUJTSXQOI-UHFFFAOYSA-N | |
Source | PubChem | |
URL | https://pubchem.ncbi.nlm.nih.gov | |
Description | Data deposited in or computed by PubChem | |
Canonical SMILES |
O=[V]=O | |
Source | PubChem | |
URL | https://pubchem.ncbi.nlm.nih.gov | |
Description | Data deposited in or computed by PubChem | |
Molecular Formula |
VO2, O2V | |
Record name | Vanadium(IV) oxide | |
Source | Wikipedia | |
URL | https://en.wikipedia.org/wiki/Vanadium(IV)_oxide | |
Description | Chemical information link to Wikipedia. | |
Source | PubChem | |
URL | https://pubchem.ncbi.nlm.nih.gov | |
Description | Data deposited in or computed by PubChem | |
DSSTOX Substance ID |
DTXSID5065194 | |
Record name | Vanadium oxide (VO2) | |
Source | EPA DSSTox | |
URL | https://comptox.epa.gov/dashboard/DTXSID5065194 | |
Description | DSSTox provides a high quality public chemistry resource for supporting improved predictive toxicology. | |
Molecular Weight |
82.940 g/mol | |
Source | PubChem | |
URL | https://pubchem.ncbi.nlm.nih.gov | |
Description | Data deposited in or computed by PubChem | |
Physical Description |
Black crystalline solid; Insoluble in water; [MSDSonline] | |
Record name | Vanadium dioxide | |
Source | Haz-Map, Information on Hazardous Chemicals and Occupational Diseases | |
URL | https://haz-map.com/Agents/8223 | |
Description | Haz-Map® is an occupational health database designed for health and safety professionals and for consumers seeking information about the adverse effects of workplace exposures to chemical and biological agents. | |
Explanation | Copyright (c) 2022 Haz-Map(R). All rights reserved. Unless otherwise indicated, all materials from Haz-Map are copyrighted by Haz-Map(R). No part of these materials, either text or image may be used for any purpose other than for personal use. Therefore, reproduction, modification, storage in a retrieval system or retransmission, in any form or by any means, electronic, mechanical or otherwise, for reasons other than personal use, is strictly prohibited without prior written permission. | |
CAS No. |
12036-21-4 | |
Record name | Vanadium dioxide | |
Source | CAS Common Chemistry | |
URL | https://commonchemistry.cas.org/detail?cas_rn=12036-21-4 | |
Description | CAS Common Chemistry is an open community resource for accessing chemical information. Nearly 500,000 chemical substances from CAS REGISTRY cover areas of community interest, including common and frequently regulated chemicals, and those relevant to high school and undergraduate chemistry classes. This chemical information, curated by our expert scientists, is provided in alignment with our mission as a division of the American Chemical Society. | |
Explanation | The data from CAS Common Chemistry is provided under a CC-BY-NC 4.0 license, unless otherwise stated. | |
Record name | Vanadium oxide (VO2) | |
Source | ChemIDplus | |
URL | https://pubchem.ncbi.nlm.nih.gov/substance/?source=chemidplus&sourceid=0012036214 | |
Description | ChemIDplus is a free, web search system that provides access to the structure and nomenclature authority files used for the identification of chemical substances cited in National Library of Medicine (NLM) databases, including the TOXNET system. | |
Record name | Vanadium oxide (VO2) | |
Source | EPA Chemicals under the TSCA | |
URL | https://www.epa.gov/chemicals-under-tsca | |
Description | EPA Chemicals under the Toxic Substances Control Act (TSCA) collection contains information on chemicals and their regulations under TSCA, including non-confidential content from the TSCA Chemical Substance Inventory and Chemical Data Reporting. | |
Record name | Vanadium oxide (VO2) | |
Source | EPA DSSTox | |
URL | https://comptox.epa.gov/dashboard/DTXSID5065194 | |
Description | DSSTox provides a high quality public chemistry resource for supporting improved predictive toxicology. | |
Record name | Vanadium dioxide | |
Source | European Chemicals Agency (ECHA) | |
URL | https://echa.europa.eu/substance-information/-/substanceinfo/100.031.661 | |
Description | The European Chemicals Agency (ECHA) is an agency of the European Union which is the driving force among regulatory authorities in implementing the EU's groundbreaking chemicals legislation for the benefit of human health and the environment as well as for innovation and competitiveness. | |
Explanation | Use of the information, documents and data from the ECHA website is subject to the terms and conditions of this Legal Notice, and subject to other binding limitations provided for under applicable law, the information, documents and data made available on the ECHA website may be reproduced, distributed and/or used, totally or in part, for non-commercial purposes provided that ECHA is acknowledged as the source: "Source: European Chemicals Agency, http://echa.europa.eu/". Such acknowledgement must be included in each copy of the material. ECHA permits and encourages organisations and individuals to create links to the ECHA website under the following cumulative conditions: Links can only be made to webpages that provide a link to the Legal Notice page. | |
Retrosynthesis Analysis
AI-Powered Synthesis Planning: Our tool employs the Template_relevance Pistachio, Template_relevance Bkms_metabolic, Template_relevance Pistachio_ringbreaker, Template_relevance Reaxys, Template_relevance Reaxys_biocatalysis model, leveraging a vast database of chemical reactions to predict feasible synthetic routes.
One-Step Synthesis Focus: Specifically designed for one-step synthesis, it provides concise and direct routes for your target compounds, streamlining the synthesis process.
Accurate Predictions: Utilizing the extensive PISTACHIO, BKMS_METABOLIC, PISTACHIO_RINGBREAKER, REAXYS, REAXYS_BIOCATALYSIS database, our tool offers high-accuracy predictions, reflecting the latest in chemical research and data.
Strategy Settings
Precursor scoring | Relevance Heuristic |
---|---|
Min. plausibility | 0.01 |
Model | Template_relevance |
Template Set | Pistachio/Bkms_metabolic/Pistachio_ringbreaker/Reaxys/Reaxys_biocatalysis |
Top-N result to add to graph | 6 |
Feasible Synthetic Routes
Q1: What makes vanadium dioxide unique compared to other materials exhibiting metal-insulator transitions?
A1: While other materials undergo IMT, VO2 stands out due to its transition temperature (around 68°C) being close to room temperature. [] This proximity to ambient conditions makes VO2 highly attractive for practical applications where temperature control is crucial.
Q2: How does the phase transition in VO2 manifest in its optical properties?
A2: The IMT in VO2 triggers a dramatic change in its optical properties, specifically a significant decrease in infrared (IR) transmittance. [] In its insulating state, VO2 is transparent to IR, while in its metallic state, it becomes reflective to IR.
Q3: What is the significance of this compound's tunable refractive index?
A3: VO2's refractive index can be precisely controlled by manipulating its temperature within the transition region. [] This tunability opens doors for applications in dynamic optical devices, such as optical switches and modulators.
Q4: How can the transition temperature of VO2 be adjusted?
A4: Doping with elements like tungsten or molybdenum is a common method to modify the IMT temperature of VO2. [, ] This doping disrupts the Peierls pairing, influencing the transition temperature to better suit specific applications.
Q5: What are some common methods for synthesizing this compound thin films?
A5: Various methods are employed for VO2 thin film synthesis, including sputtering, [, , , ] sol-gel dip coating, [] atomic layer deposition (ALD), [] and rapid thermal oxidation. [] The choice of method depends on the desired film quality, scalability, and cost-effectiveness.
Q6: Why is controlling the stoichiometry crucial during this compound thin film preparation?
A6: Maintaining precise stoichiometry is vital to achieve the desired phase transition properties in VO2 films. [] Even slight deviations can significantly impact the transition temperature, hysteresis width, and optical modulation depth.
Q7: How can selective deposition of this compound be achieved?
A7: Selective area deposition of VO2 can be achieved using techniques like metal-organic chemical vapor deposition (MOCVD). By carefully controlling the precursor flow and substrate conditions, VO2 growth can be confined to specific areas, enabling the fabrication of patterned structures. []
Q8: What challenges arise in fabricating this compound nanostructures, and how are they addressed?
A8: Fabricating VO2 nanostructures requires precise control over dimensions and morphology. Top-down approaches, like nanomachining the substrate before or after film deposition, have been successful in creating VO2 nanostructures with dimensions down to 100 nm, preserving their phase transition behavior. []
Q9: What makes this compound a promising material for infrared camouflage?
A9: VO2's ability to transition between states with drastically different IR reflectivity makes it suitable for active infrared camouflage. [] By controlling the temperature and thus the phase of VO2, the emissivity of the material can be modulated, enabling dynamic camouflage against IR detection.
Q10: How is this compound employed in terahertz (THz) wave manipulation devices?
A10: VO2's phase transition significantly impacts its interaction with THz waves. [, , , , ] This property is exploited in devices like THz modulators, absorbers, and polarization converters. The transition between insulating and metallic states allows for dynamic control over the transmission, reflection, and polarization of THz radiation.
Q11: How can this compound contribute to energy-saving applications?
A11: Thermochromic smart windows utilize VO2's ability to modulate IR transmission based on temperature. [] These windows can potentially reduce energy consumption by controlling the amount of solar heat entering buildings, adapting to changing weather conditions.
Q12: What are the advantages of using this compound in optical switches compared to other materials?
A12: VO2-based optical switches offer ultrafast switching speeds, operating on a femtosecond scale. [] This speed, combined with the significant change in optical properties during the phase transition, makes VO2 attractive for high-speed optical communication and data processing applications.
Q13: How does the integration of this compound with metasurfaces enhance device functionality?
A13: Integrating VO2 with metasurfaces allows for dynamic control over the properties of electromagnetic waves. [, , , , , ] The phase transition of VO2 can be used to tune the resonance frequency, absorption, and polarization conversion capabilities of metasurfaces, leading to reconfigurable and multifunctional devices.
Q14: What insights do computational studies offer into the behavior of this compound?
A14: Computational methods, like density functional theory (DFT), help investigate the electronic structure and phase transition mechanism in VO2. [, ] These studies contribute to a deeper understanding of the interplay between structural, electronic, and optical properties, guiding the development of tailored VO2 materials.
Disclaimer and Information on In-Vitro Research Products
Please be aware that all articles and product information presented on BenchChem are intended solely for informational purposes. The products available for purchase on BenchChem are specifically designed for in-vitro studies, which are conducted outside of living organisms. In-vitro studies, derived from the Latin term "in glass," involve experiments performed in controlled laboratory settings using cells or tissues. It is important to note that these products are not categorized as medicines or drugs, and they have not received approval from the FDA for the prevention, treatment, or cure of any medical condition, ailment, or disease. We must emphasize that any form of bodily introduction of these products into humans or animals is strictly prohibited by law. It is essential to adhere to these guidelines to ensure compliance with legal and ethical standards in research and experimentation.