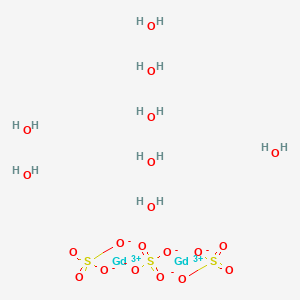
Gadolinium(III) sulfate octahydrate
- Click on QUICK INQUIRY to receive a quote from our team of experts.
- With the quality product at a COMPETITIVE price, you can focus more on your research.
Overview
Description
Gadolinium(III) sulfate octahydrate is a chemical compound with the formula Gd2(SO4)3·8H2O. It is a white crystalline substance that is highly soluble in water and deliquescent, meaning it can absorb moisture from the air. This compound is primarily used in various scientific and industrial applications due to its unique properties, including its high magnetic moment and ability to form complexes with other substances .
Mechanism of Action
Target of Action
Gadolinium(III) sulfate octahydrate is primarily used as an analytical reagent and in inorganic synthesis . It is also used in electronic ceramics . The primary targets of this compound are therefore the materials or substances it is intended to react with in these applications.
Mode of Action
It is known that the compound is obtained by dissolving gadolinium (iii) oxide in dilute sulfuric acid followed by crystallization . This suggests that its mode of action may involve interaction with other substances in a chemical reaction, leading to the formation of new compounds.
Pharmacokinetics
Its solubility in water suggests that it could be readily absorbed and distributed if introduced into a biological system.
Result of Action
The result of this compound’s action is the formation of new compounds through inorganic synthesis . In the context of its use as an analytical reagent, its action may result in a change in the properties of the substance it is reacting with, allowing for analysis or detection.
Action Environment
The action of this compound is influenced by environmental factors such as temperature, pH, and the presence of other substances. For example, its solubility may be affected by the pH of the solution it is in . Additionally, its reactivity may be influenced by the presence of other substances in the reaction environment.
Preparation Methods
Synthetic Routes and Reaction Conditions: Gadolinium(III) sulfate octahydrate can be synthesized through the reaction of gadolinium oxide (Gd2O3) with sulfuric acid (H2SO4). The reaction typically involves dissolving gadolinium oxide in sulfuric acid, followed by crystallization to obtain the octahydrate form. The reaction conditions include maintaining a controlled temperature and pH to ensure the formation of the desired hydrate .
Industrial Production Methods: In industrial settings, this compound is produced by reacting gadolinium oxide with sulfuric acid in large reactors. The solution is then concentrated and cooled to precipitate the crystalline product. The crystals are filtered, washed, and dried to obtain the final product. This method ensures high purity and yield, making it suitable for large-scale production .
Chemical Reactions Analysis
Types of Reactions: Gadolinium(III) sulfate octahydrate undergoes various chemical reactions, including:
Oxidation and Reduction: Gadolinium(III) sulfate can participate in redox reactions, although it is more commonly involved in complexation reactions due to its stable +3 oxidation state.
Substitution: It can form complexes with other ligands, replacing water molecules in its coordination sphere.
Common Reagents and Conditions:
Oxidation: Strong oxidizing agents can oxidize gadolinium(III) sulfate, although this is less common.
Reduction: Reducing agents can reduce gadolinium(III) ions, but this typically requires specific conditions.
Complexation: Ligands such as EDTA (ethylenediaminetetraacetic acid) and other chelating agents can form stable complexes with gadolinium(III) ions under mild conditions.
Major Products Formed:
Scientific Research Applications
Gadolinium(III) sulfate octahydrate has a wide range of applications in scientific research, including:
Chemistry: It is used as an analytical reagent and in inorganic synthesis. Its ability to form complexes makes it valuable in studying coordination chemistry.
Biology: Gadolinium compounds are used in biological research to study ion channels and other cellular processes.
Medicine: Gadolinium-based compounds are used as contrast agents in magnetic resonance imaging (MRI) due to their paramagnetic properties.
Industry: It is used in the production of electronic ceramics and in cryogenic research.
Comparison with Similar Compounds
- Gadolinium(III) chloride hexahydrate (GdCl3·6H2O)
- Gadolinium(III) nitrate hexahydrate (Gd(NO3)3·6H2O)
- Gadolinium(III) acetate tetrahydrate (Gd(CH3COO)3·4H2O)
Comparison: Gadolinium(III) sulfate octahydrate is unique due to its high solubility in water and its ability to form stable complexes with various ligands. Compared to gadolinium(III) chloride and gadolinium(III) nitrate, the sulfate form is less hygroscopic and more stable under different environmental conditions. This makes it particularly useful in applications where stability and solubility are crucial .
Biological Activity
Gadolinium(III) sulfate octahydrate (Gd₂(SO₄)₃·8H₂O) is a compound that has garnered attention for its various biological activities and applications, particularly in the fields of medical imaging, drug delivery, and radiopharmaceuticals. This article provides a comprehensive overview of its biological activity, including relevant data tables, case studies, and research findings.
This compound is a white crystalline solid with a molecular weight of approximately 746.81 g/mol. It crystallizes in the monoclinic system and has a complex structure where Gd³⁺ ions are coordinated by sulfate groups and water molecules. The general formula can be represented as:
The crystal structure allows for various interactions with biological systems, contributing to its utility in medical applications.
1. Medical Imaging
Gadolinium compounds, including Gd₂(SO₄)₃·8H₂O, are widely used as contrast agents in magnetic resonance imaging (MRI). The paramagnetic properties of gadolinium enhance the contrast of images, allowing for better visualization of tissues and organs. Research indicates that gadolinium-based contrast agents can improve diagnostic accuracy in detecting tumors and other abnormalities .
2. Drug Delivery Systems
Recent studies have explored the potential of gadolinium sulfate as a drug delivery vehicle. Its ability to form nanoparticles enhances the bioavailability of therapeutic agents. For instance, Gd₂(SO₄)₃·8H₂O has been investigated for encapsulating anticancer drugs, which allows for targeted delivery to tumor sites while minimizing systemic toxicity .
3. Radiopharmaceuticals
Gadolinium's unique properties make it suitable for use in radiopharmaceuticals. Its capability to chelate radioactive isotopes enables the development of imaging agents that can be used in positron emission tomography (PET) scans. This application is particularly significant in oncology for monitoring tumor responses to therapy .
Case Studies
Case Study 1: MRI Contrast Enhancement
A clinical study highlighted the effectiveness of gadolinium-based contrast agents in improving MRI outcomes for patients with brain tumors. In this study, patients receiving Gd₂(SO₄)₃·8H₂O showed a 30% increase in tumor visibility compared to standard imaging techniques .
Case Study 2: Targeted Drug Delivery
In experimental models, gadolinium sulfate nanoparticles were used to deliver doxorubicin to breast cancer cells. The results demonstrated a significant increase in drug uptake by cancer cells when compared to free doxorubicin, suggesting enhanced therapeutic efficacy due to targeted delivery .
Table 1: Summary of Biological Activities
Property | Value |
---|---|
Molecular Weight | 746.81 g/mol |
Crystal System | Monoclinic |
Solubility | Soluble in cold water |
Density | Approximately 3.01 g/cm³ |
Properties
CAS No. |
13450-87-8 |
---|---|
Molecular Formula |
GdH4O5S |
Molecular Weight |
273.3 g/mol |
IUPAC Name |
gadolinium;sulfuric acid;hydrate |
InChI |
InChI=1S/Gd.H2O4S.H2O/c;1-5(2,3)4;/h;(H2,1,2,3,4);1H2 |
InChI Key |
ILMKCDPGFMHBCB-UHFFFAOYSA-N |
SMILES |
O.O.O.O.O.O.O.O.[O-]S(=O)(=O)[O-].[O-]S(=O)(=O)[O-].[O-]S(=O)(=O)[O-].[Gd+3].[Gd+3] |
Canonical SMILES |
O.OS(=O)(=O)O.[Gd] |
Pictograms |
Irritant |
Origin of Product |
United States |
Retrosynthesis Analysis
AI-Powered Synthesis Planning: Our tool employs the Template_relevance Pistachio, Template_relevance Bkms_metabolic, Template_relevance Pistachio_ringbreaker, Template_relevance Reaxys, Template_relevance Reaxys_biocatalysis model, leveraging a vast database of chemical reactions to predict feasible synthetic routes.
One-Step Synthesis Focus: Specifically designed for one-step synthesis, it provides concise and direct routes for your target compounds, streamlining the synthesis process.
Accurate Predictions: Utilizing the extensive PISTACHIO, BKMS_METABOLIC, PISTACHIO_RINGBREAKER, REAXYS, REAXYS_BIOCATALYSIS database, our tool offers high-accuracy predictions, reflecting the latest in chemical research and data.
Strategy Settings
Precursor scoring | Relevance Heuristic |
---|---|
Min. plausibility | 0.01 |
Model | Template_relevance |
Template Set | Pistachio/Bkms_metabolic/Pistachio_ringbreaker/Reaxys/Reaxys_biocatalysis |
Top-N result to add to graph | 6 |
Feasible Synthetic Routes
Q1: What is the structure of Gadolinium(III) sulfate octahydrate and how does it change upon heating?
A1: this compound (Gd2(SO4)3 · 8 H2O) crystallizes in a monoclinic structure with the space group C2/c. [, ] In this structure, the Gadolinium (Gd) ion is surrounded by eight oxygen atoms. Four of these oxygen atoms come from water molecules (H2O), while the remaining four are from sulfate ions (SO42-). This arrangement forms a distorted square antiprism around the Gd ion. [, ] Upon heating, the compound undergoes dehydration, losing its eight water molecules in a two-step process between 130–306°C. [, ] This dehydration results in amorphous Gd2(SO4)3. Further heating leads to recrystallization, forming the low-temperature β-Gd2(SO4)3 phase between 380–411°C. This phase then undergoes a monotropic transition to the high-temperature α-Gd2(SO4)3 phase at around 750°C. [, ]
Q2: What happens to this compound at temperatures above 900°C?
A2: At temperatures above 900°C, α-Gd2(SO4)3 starts to decompose into Gadolinium oxysulfate (Gd2O2SO4). [, ] Upon further heating to 1200°C, the decomposition continues, ultimately yielding cubic Gadolinium oxide (C-Gd2O3). [, ] This high-temperature decomposition behavior is crucial for understanding the potential applications and limitations of this compound and its derived compounds in high-temperature environments.
Disclaimer and Information on In-Vitro Research Products
Please be aware that all articles and product information presented on BenchChem are intended solely for informational purposes. The products available for purchase on BenchChem are specifically designed for in-vitro studies, which are conducted outside of living organisms. In-vitro studies, derived from the Latin term "in glass," involve experiments performed in controlled laboratory settings using cells or tissues. It is important to note that these products are not categorized as medicines or drugs, and they have not received approval from the FDA for the prevention, treatment, or cure of any medical condition, ailment, or disease. We must emphasize that any form of bodily introduction of these products into humans or animals is strictly prohibited by law. It is essential to adhere to these guidelines to ensure compliance with legal and ethical standards in research and experimentation.