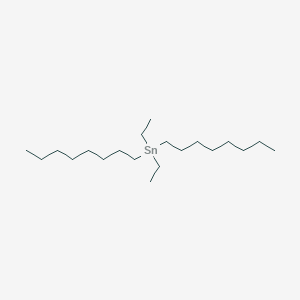
Stannane, diethyl-, di(n-octyl)-
Overview
Description
Stannane, diethyl-, di(n-octyl)- is an organotin compound with the molecular formula C₁₂H₂₈Sn, featuring two ethyl (C₂H₅) and two linear n-octyl (C₈H₁₇) groups bonded to a central tin atom. Organotin compounds are widely utilized as stabilizers in plastics, catalysts in industrial processes, and biocides, though their applications are increasingly regulated due to environmental and toxicity concerns .
Synthesis:
The synthesis of dialkyltin compounds typically involves chlorination and subsequent alkylation. For instance, describes the preparation of dichlorinated stannanes via chlorination of precursor compounds (>95% yield), followed by recrystallization from hexane and dichloromethane (DCM) to achieve high-purity crystalline solids . While direct evidence for the synthesis of diethyl-di(n-octyl)stannane is absent, analogous methods using Grignard reagents or alkyl lithium agents to substitute chloride ligands with ethyl and n-octyl groups are plausible.
Preparation Methods
Synthetic Routes and Reaction Conditions: The synthesis of stannane, diethyl-, di(n-octyl)- typically involves the reaction of diethylstannane with n-octyl halides under controlled conditions. The reaction is usually carried out in the presence of a catalyst to facilitate the formation of the desired product. The reaction conditions, such as temperature and pressure, are carefully monitored to ensure high yield and purity of the compound.
Industrial Production Methods: In an industrial setting, the production of stannane, diethyl-, di(n-octyl)- involves large-scale reactions using similar methods as in the laboratory. The process is optimized for efficiency and cost-effectiveness, often employing continuous flow reactors and advanced purification techniques to obtain the final product.
Chemical Reactions Analysis
Types of Reactions: Stannane, diethyl-, di(n-octyl)- undergoes various chemical reactions, including:
Oxidation: The compound can be oxidized to form organotin oxides or hydroxides.
Reduction: It can be reduced to form lower oxidation state organotin compounds.
Substitution: The organic groups attached to the tin atom can be substituted with other groups through nucleophilic substitution reactions.
Common Reagents and Conditions:
Oxidation: Common oxidizing agents include hydrogen peroxide and oxygen.
Reduction: Reducing agents such as lithium aluminum hydride are used.
Substitution: Nucleophiles like halides or alkoxides are employed in substitution reactions.
Major Products Formed:
Oxidation: Organotin oxides and hydroxides.
Reduction: Lower oxidation state organotin compounds.
Substitution: New organotin compounds with different organic groups.
Scientific Research Applications
2.1. Plastics and Polymers
Stannane, diethyl-, di(n-octyl)- is utilized as a stabilizer and process regulator in the manufacture of plastics. It helps enhance the thermal stability and longevity of polymer products, particularly in PVC formulations. The compound can improve the mechanical properties of plastics, making them suitable for various applications including construction materials and consumer goods .
2.2. Adhesives and Sealants
In adhesives and sealants, this stannane compound acts as a catalyst that promotes cross-linking reactions, which are essential for achieving strong bonds in diverse materials. Its effectiveness at low concentrations makes it a preferred choice in industrial applications .
3.1. Biodegradation Studies
Research indicates that organotin compounds, including diethyl-, di(n-octyl)- stannane, can undergo hydrolysis to release toxic byproducts such as 2-ethylhexanoic acid (2-EHA) . Studies focus on the environmental impact of these compounds, particularly their toxicity to aquatic life and potential bioaccumulation in ecosystems .
3.2. Analytical Methods for Detection
The detection and quantification of organotin compounds in environmental samples have been developed using advanced analytical techniques such as gas chromatography coupled with mass spectrometry (GC-MS). These methods are crucial for monitoring compliance with environmental regulations regarding organotin levels in water bodies .
4.1. Toxicological Profiles
Toxicological assessments indicate that stannane compounds exhibit low acute toxicity but can cause systemic effects upon prolonged exposure. Specific studies have reported that repeated exposure may lead to immunotoxicity and developmental toxicity in laboratory animals .
4.2. Regulatory Frameworks
The European Chemicals Agency (ECHA) has classified certain organotin compounds as hazardous due to their potential to cause harm to human health and the environment. Regulatory measures are in place to limit their use in consumer products, particularly those intended for children .
Case Studies
Mechanism of Action
The mechanism by which stannane, diethyl-, di(n-octyl)- exerts its effects involves the interaction of the tin atom with various molecular targets. The tin atom can form bonds with different functional groups, influencing the reactivity and stability of the compound. The pathways involved include coordination with ligands and participation in redox reactions.
Comparison with Similar Compounds
Physical Properties :
- Molecular Weight : ~347.1 g/mol (estimated based on alkyl chain contributions).
- Physical State : Likely a semi-solid or low-melting solid at room temperature, similar to dihydridostannanes isolated as white semi-solids in .
- Solubility: Expected to be soluble in nonpolar solvents like hexane and DCM, consistent with purification methods for related compounds .
Comparison with Similar Organotin Compounds
Structural and Functional Comparisons
The table below compares diethyl-di(n-octyl)stannane with other organotin compounds in terms of structure, properties, and applications:
Key Observations :
- Alkyl Chain Length: Longer n-octyl groups in diethyl-di(n-octyl)stannane may reduce volatility and increase hydrophobicity compared to shorter-chain analogs like dibutyltin compounds.
- Branching vs. Linear Chains : highlights that linear alkyl chains (e.g., di(n-octyl) sebacate) have higher melting points (+18.6°C) than branched isomers (-55°C), suggesting diethyl-di(n-octyl)stannane may exhibit similar solid-state stability .
Environmental and Toxicological Profiles
- Toxicity: Shorter-chain organotins (e.g., tributyltin) are highly toxic, disrupting endocrine systems in aquatic organisms. Longer chains, as in diethyl-di(n-octyl)stannane, may exhibit reduced bioavailability and slower degradation, leading to bioaccumulation .
- Regulatory Status : Compounds like tributyltin methacrylate are restricted under international regulations (e.g., International Maritime Organization). Diethyl-di(n-octyl)stannane, if commercialized, would likely face scrutiny under REACH or similar frameworks .
Biological Activity
Stannane, diethyl-, di(n-octyl)- is an organotin compound that has garnered attention in various fields, particularly in organic synthesis and biological research. This article delves into its biological activity, focusing on its potential applications and toxicity, supported by data tables and relevant case studies.
Chemical Structure and Properties
Stannane, diethyl-, di(n-octyl)- has the molecular formula and features a tin atom bonded to two ethyl groups and two n-octyl groups. Its chemical structure allows for unique interactions with biological systems, influencing its activity as a reagent in organic synthesis and its potential biological effects.
Biological Activity
Antifungal and Antibacterial Properties
Organotin compounds, including stannane, diethyl-, di(n-octyl)-, have been investigated for their antifungal and antibacterial properties. Studies indicate that these compounds can disrupt cellular membranes of fungi and bacteria, leading to cell death. For instance, organotin compounds have shown efficacy against various fungal strains, suggesting their potential use in agricultural applications as antifungal agents .
Anticancer Activity
Research has demonstrated that stannane, diethyl-, di(n-octyl)- exhibits anticancer properties. In vitro studies have assessed its antiproliferative effects on cancer cell lines such as HCT-116 (colon cancer), MCF-7 (breast cancer), and A-549 (lung cancer). The results indicated that certain organotin derivatives can induce apoptosis in cancer cells, potentially through mechanisms involving cell cycle arrest at the G2/M phase .
The biological activity of stannane, diethyl-, di(n-octyl)- is attributed to several mechanisms:
- Membrane Disruption : The hydrophobic nature of the n-octyl groups facilitates insertion into lipid bilayers, causing membrane destabilization.
- Reactive Oxygen Species (ROS) Generation : Some studies suggest that organotin compounds can induce oxidative stress within cells, leading to damage that triggers apoptosis.
- Enzyme Inhibition : Organotin compounds may inhibit key enzymes involved in cellular metabolism or signaling pathways relevant to cancer progression .
Toxicity and Safety Concerns
Despite their potential therapeutic applications, organotin compounds are associated with significant toxicity. The following table summarizes findings related to the toxicity of stannane, diethyl-, di(n-octyl)- and similar organotin compounds:
Compound | Toxicity Level | Key Findings |
---|---|---|
Stannane, diethyl-, di(n-octyl)- | Moderate | Induces apoptosis in cancer cells; potential for membrane disruption |
Dibutyltin dichloride | High | Associated with reproductive toxicity and immunotoxicity |
Dimethyltin | Low | Limited genotoxic effects; no significant carcinogenicity observed |
Studies have shown that repeated exposure to organotin compounds may lead to systemic health effects such as reproductive toxicity and neurotoxicity . For example, dibutyltin compounds exhibited significant adverse effects on fertility in animal studies.
Case Studies
- Anticancer Efficacy : A study investigating the antiproliferative effects of various organotin compounds found that stannane derivatives significantly reduced cell viability in human cancer cell lines compared to controls. The IC50 values indicated a promising therapeutic index for further development .
- Toxicological Assessment : An evaluation of dibutyltin compounds highlighted their reproductive toxicity, which raises concerns about the safety of using similar organotin compounds in therapeutic settings. The study emphasized the need for thorough risk assessments before clinical applications .
Q & A
Q. Basic: What are the established synthetic routes for diethyl-di(n-octyl)stannane, and how can reaction conditions be optimized for high purity?
Methodological Answer:
Diethyl-di(n-octyl)stannane is typically synthesized via transmetallation or alkylation of tin halides. A common approach involves reacting di(n-octyl)tin dichloride (precursor) with ethyl Grignard reagents (e.g., ethylmagnesium bromide) in anhydrous ether under inert atmosphere. Key optimization steps include:
- Molar Ratio: Maintain a 2:1 molar ratio of Grignard reagent to tin dichloride to ensure complete substitution of chloride ligands .
- Temperature Control: Gradual addition of the Grignard reagent at 0–5°C minimizes side reactions (e.g., disproportionation) .
- Purification: Post-reaction hydrolysis followed by vacuum distillation or column chromatography (using silica gel and non-polar solvents) isolates the product. Purity can be verified via Sn NMR, where a single resonance at δ ≈ -200 ppm confirms a tetraalkyltin structure .
Q. Basic: Which spectroscopic techniques are most effective for characterizing diethyl-di(n-octyl)stannane, and what spectral features confirm its structure?
Methodological Answer:
- Sn NMR: The most definitive technique. A singlet in the range δ -180 to -220 ppm confirms a symmetrical tetraalkyltin structure. For diethyl-di(n-octyl)stannane, splitting patterns (if any) arise from inequivalent alkyl groups .
- FT-IR: Absence of Sn-Cl stretches (~350 cm) and presence of Sn-C stretches (~450–550 cm) validate successful alkylation.
- Mass Spectrometry (MS): High-resolution MS shows molecular ion peaks matching the molecular formula (calc. ~435.3 g/mol). Isotopic patterns for tin (e.g., Sn, Sn) further confirm the structure.
Table 1: Key Spectral Data
Technique | Diagnostic Feature | Reference |
---|---|---|
Sn NMR | δ ≈ -200 ppm (singlet) | |
FT-IR | Sn-C stretch at ~500 cm | |
HRMS | [M] at m/z 435.3 (CHSn) |
Q. Advanced: How does the steric environment of diethyl-di(n-octyl)stannane influence its reactivity in cross-coupling reactions compared to less substituted stannanes?
Methodological Answer:
The bulky n-octyl and ethyl groups create a sterically hindered tin center, reducing its efficacy in Stille couplings compared to trimethyl- or tributylstannanes. Key considerations:
- Reactivity Trade-offs: While steric bulk slows transmetallation kinetics, it enhances stability against hydrolysis and oxidation.
- Experimental Design: Compare reaction rates using kinetic studies (e.g., monitoring via Sn NMR) with less hindered analogs. Adjust ligands (e.g., Pd(PPh)) to facilitate oxidative addition .
- Case Study: In Suzuki-Miyaura couplings, diethyl-di(n-octyl)stannane may require higher temperatures (80–100°C) and prolonged reaction times (>24 h) for comparable yields.
Q. Advanced: What are the key considerations for analyzing the thermal stability and decomposition pathways of diethyl-di(n-octyl)stannane under inert and oxidative conditions?
Methodological Answer:
- Thermogravimetric Analysis (TGA): Conduct under N (inert) and O (oxidative) atmospheres. Decomposition onset temperatures (typically >200°C under N) indicate stability.
- GC-MS for Byproducts: Under oxidative conditions, expect SnO residue and volatile alkanes (e.g., octane, ethane). Inert conditions may yield tin carbides or oligomeric species .
- Safety Note: Decomposition releases toxic gases (e.g., CO, NO). Use fume hoods and inline scrubbers during pyrolysis experiments .
Q. Advanced: How can computational methods (e.g., DFT) be applied to predict the coordination behavior and catalytic potential of diethyl-di(n-octyl)stannane?
Methodological Answer:
- DFT Calculations: Optimize geometry using B3LYP/def2-TZVP to assess bond angles and electron density at the tin center. High electron density at Sn correlates with nucleophilic reactivity.
- Ligand Displacement Studies: Simulate interactions with Lewis acids (e.g., B(CF)) to predict adduct formation. Compare activation energies for Sn-C bond cleavage with experimental TGA data.
- Catalytic Screening: Use docking simulations to evaluate potential as a catalyst in esterification or hydrostannylation, guided by steric maps of the alkyl groups .
Q. Basic: What are the recommended safety protocols for handling diethyl-di(n-ooctyl)stannane in laboratory settings, based on its toxicity profile?
Methodological Answer:
- PPE: Wear nitrile gloves, lab coats, and safety goggles. Use closed systems to avoid inhalation of vapors/aerosols .
- Ventilation: Conduct experiments in fume hoods with >0.5 m/s face velocity.
- Spill Management: Absorb with vermiculite, neutralize with 10% KOH, and dispose as hazardous waste. Avoid water to prevent tin oxide formation .
- Toxicity Data: LD (oral, rat): ~500 mg/kg; chronic exposure risks include neurotoxicity and organ damage .
Q. Advanced: What experimental approaches can resolve discrepancies in reported kinetic data for transmetallation reactions involving diethyl-di(n-octyl)stannane?
Methodological Answer:
- Isotopic Labeling: Use C-labeled substrates to track Sn-C bond cleavage via NMR or MS.
- In Situ Monitoring: Employ stopped-flow UV-Vis or Raman spectroscopy to capture transient intermediates.
- Control for Impurities: Pre-purify stannane via recrystallization and validate via Sn NMR (>98% purity). Replicate conflicting studies under identical conditions (solvent, temperature, catalyst loading) .
Properties
IUPAC Name |
diethyl(dioctyl)stannane | |
---|---|---|
Source | PubChem | |
URL | https://pubchem.ncbi.nlm.nih.gov | |
Description | Data deposited in or computed by PubChem | |
InChI |
InChI=1S/2C8H17.2C2H5.Sn/c2*1-3-5-7-8-6-4-2;2*1-2;/h2*1,3-8H2,2H3;2*1H2,2H3; | |
Source | PubChem | |
URL | https://pubchem.ncbi.nlm.nih.gov | |
Description | Data deposited in or computed by PubChem | |
InChI Key |
MNJUXQIYWVMUHY-UHFFFAOYSA-N | |
Source | PubChem | |
URL | https://pubchem.ncbi.nlm.nih.gov | |
Description | Data deposited in or computed by PubChem | |
Canonical SMILES |
CCCCCCCC[Sn](CC)(CC)CCCCCCCC | |
Source | PubChem | |
URL | https://pubchem.ncbi.nlm.nih.gov | |
Description | Data deposited in or computed by PubChem | |
Molecular Formula |
C20H44Sn | |
Source | PubChem | |
URL | https://pubchem.ncbi.nlm.nih.gov | |
Description | Data deposited in or computed by PubChem | |
Molecular Weight |
403.3 g/mol | |
Source | PubChem | |
URL | https://pubchem.ncbi.nlm.nih.gov | |
Description | Data deposited in or computed by PubChem | |
Disclaimer and Information on In-Vitro Research Products
Please be aware that all articles and product information presented on BenchChem are intended solely for informational purposes. The products available for purchase on BenchChem are specifically designed for in-vitro studies, which are conducted outside of living organisms. In-vitro studies, derived from the Latin term "in glass," involve experiments performed in controlled laboratory settings using cells or tissues. It is important to note that these products are not categorized as medicines or drugs, and they have not received approval from the FDA for the prevention, treatment, or cure of any medical condition, ailment, or disease. We must emphasize that any form of bodily introduction of these products into humans or animals is strictly prohibited by law. It is essential to adhere to these guidelines to ensure compliance with legal and ethical standards in research and experimentation.