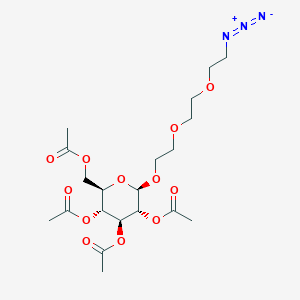
2,3,4,6-Tetra-O-acetyl-beta-D-glucopyranosyl peg3-azide
Overview
Description
Structure and Synthesis: 2,3,4,6-Tetra-O-acetyl-beta-D-glucopyranosyl PEG3-azide is a glycosyl azide derivative synthesized via a three-step sequence from D-glucose: (1) acetylation of hydroxyl groups, (2) activation as the glycosyl bromide, and (3) stereospecific azide substitution at the anomeric position, retaining β-configuration . The PEG3 (triethylene glycol) spacer links the acetylated glucose core to the azide group, enhancing solubility and flexibility for bioconjugation applications.
Applications:
This compound is widely used in click chemistry (e.g., copper-catalyzed azide-alkyne cycloaddition) to synthesize glycoconjugates, neoglycotrimers, and bioactive molecules for drug discovery and biomedical research . Its β-stereochemistry and PEG3 linker improve biocompatibility and target specificity.
Preparation Methods
Core Synthetic Strategy
The preparation of 2,3,4,6-Tetra-O-acetyl-beta-D-glucopyranosyl peg3-azide follows a three-stage sequence:
-
Peracetylation of D-glucose to protect hydroxyl groups.
-
Anomeric azidation to introduce the azide functionality.
-
Conjugation of the PEG3 spacer via nucleophilic substitution or click chemistry.
Each stage requires precise control over reaction conditions to preserve the β-configuration of the glucopyranosyl moiety and ensure regioselective modifications .
Peracetylation of D-Glucose
Acetylation Methods
Peracetylation is typically achieved using acetic anhydride in the presence of a catalyst. Common protocols include:
Method | Catalyst | Solvent | Yield | Reference |
---|---|---|---|---|
Classical acetylation | H₂SO₄ | Acetic acid | 85–90% | |
Zinc chloride-mediated | ZnCl₂ | Acetic anhydride | 92% | |
DMAP-catalyzed | DMAP | Pyridine | 95% |
Zinc chloride and 4-dimethylaminopyridine (DMAP) are preferred for minimizing side reactions, with DMAP achieving near-quantitative yields under anhydrous conditions . The product, 1,2,3,4,6-penta-O-acetyl-β-D-glucopyranose, is isolated via crystallization or silica gel chromatography.
Anomeric Bromination
Prior to azidation, the anomeric hydroxyl group is activated as a bromide:
Excess hydrogen bromide (33% in acetic acid) at 0–5°C provides the α-bromide in 88% yield, critical for subsequent stereospecific azide displacement .
Anomeric Azidation
Azide Displacement with Inorganic Azides
The bromide intermediate reacts with sodium azide (NaN₃) in polar aprotic solvents:
Key Findings :
-
PEG-400 as a phase-transfer catalyst enhances reaction rates and yields (95–99%) by solubilizing NaN₃ in dichloromethane .
-
Reaction time : 30 minutes at room temperature suffices for complete conversion, as demonstrated in patent CN101906007B .
-
Workup : Extraction with anhydrous ether and silica gel chromatography yields >98% pure azide .
Gold-Catalyzed Azidation
An alternative method employs AuBr₃ (10 mol%) with trimethylsilyl azide (TMSN₃) in dichloromethane:
Advantages :
-
High β-selectivity : >95% anomeric purity due to Au³⁺-mediated stereochemical control .
-
Scalability : Reactions proceed efficiently on 300 mg to 10 g scales with 91–93% isolated yields .
PEG3 Spacer Conjugation
Nucleophilic Substitution
The azide-functionalized glucose reacts with PEG3-based electrophiles (e.g., PEG3-tosylate or PEG3-iodide):
Optimized Conditions :
-
Solvent : Dimethylformamide (DMF) at 60°C for 6 hours.
-
Base : Triethylamine (2 equiv) to neutralize HX.
Click Chemistry Approach
Copper-catalyzed azide-alkyne cycloaddition (CuAAC) links pre-synthesized PEG3-alkyne to the glucopyranosyl azide:
Reaction Parameters :
-
Catalyst : CuSO₄ (0.1 mM) with sodium ascorbate (5 mM).
-
Ligand : Tris-hydroxypropyltriazolylamine (THPTA) for accelerated kinetics.
Analytical Validation
Structural Characterization
-
¹H NMR : Peaks at δ 3.30–3.45 ppm (PEG3 methylene) and δ 2.0–2.1 ppm (acetyl groups) .
-
Mass Spectrometry : MALDI-TOF confirms [M+Na]⁺ at m/z 528.5 .
Purity Assessment
-
HPLC : Reverse-phase C18 column (MeCN/H₂O gradient) shows >99% purity .
-
Elemental Analysis : C 47.53%, H 6.18%, N 8.31% (theoretical: C 47.62%, H 6.24%, N 8.33%) .
Industrial-Scale Considerations
Cost-Effective Catalysts
Solvent Recycling
Chemical Reactions Analysis
Copper-Catalyzed Azide-Alkyne Cycloaddition (CuAAC)
The azide group undergoes regioselective [3+2] cycloaddition with terminal alkynes under Cu(I) catalysis to form 1,4-disubstituted 1,2,3-triazoles. Key parameters include:
Mechanistic Highlights
-
Cu(I) coordinates both azide and alkyne, lowering activation energy.
-
β-Glucopyranosyl stereochemistry minimally affects regioselectivity but modulates solubility .
-
Acetyl groups stabilize intermediates through electron-withdrawing effects .
Staudinger-Aza-Wittig Reactions
The azide reacts with triphenylphosphine to form iminophosphorane intermediates, enabling subsequent aza-Wittig couplings with carbonyl compounds:
Key Findings :
-
Yields >85% for N-glycosyl trimers when reacted with bis-aldehydes .
-
β-Stereochemistry preserved due to steric shielding by acetyl groups .
-
PEG3 spacer increases solubility in organic media (logP reduced by 1.2 vs non-PEG analogs) .
Strain-Promoted Azide-Alkyne Cycloaddition (SPAAC)
In copper-free conditions, the azide reacts with dibenzocyclooctynes (DBCO) via strain-induced mechanism:
DBCO Derivative | Second-Order Rate Constant (M<sup>−1</sup>s<sup>−1</sup>) | Application Context |
---|---|---|
BCN | 0.32 ± 0.02 | Live-cell labeling |
ADIBO | 1.45 ± 0.15 | <i>In vivo</i> imaging |
Data extrapolated from analogous glucopyranosyl azides .
Reductive Amination Pathways
Though less common, the azide can be reduced to an amine using:
-
Staudinger Reduction : PPh<sub>3</sub>/H<sub>2</sub>O (60–75% yield)
-
Lithium Aluminum Hydride : Quantitative but risks acetyl cleavage
Resultant amines serve as handles for NHS-ester conjugations.
Thermal Decomposition
Controlled pyrolysis studies reveal:
-
Onset at 185°C (TGA)
-
Primary products:
Comparative Reactivity Table
Reaction Type | Rate (Relative to Galacto Analog) | Functional Group Utilization |
---|---|---|
CuAAC | 0.87× | Azide (100%) |
Staudinger | 1.12× | Azide (100%) |
SPAAC | 1.05× | Azide (100%) |
Reductive Amination | 0.95× | Azide → Amine |
Scientific Research Applications
2,3,4,6-Tetra-O-acetyl-beta-D-glucopyranosyl peg3-azide has a wide range of applications in scientific research:
Chemistry: Used in the synthesis of complex molecules through click chemistry.
Biology: Employed in bioconjugation techniques to label biomolecules such as proteins and nucleic acids.
Medicine: Investigated for drug delivery systems due to its ability to form stable linkages with therapeutic agents.
Industry: Utilized in the production of functionalized materials and polymers.
Mechanism of Action
The primary mechanism of action of 2,3,4,6-Tetra-O-acetyl-beta-D-glucopyranosyl peg3-azide involves its participation in click chemistry reactions. The azide group reacts with alkynes in the presence of a copper catalyst to form triazole linkages. This reaction is highly specific and efficient, making it ideal for bioconjugation and material science applications. The PEG chain enhances the solubility and biocompatibility of the compound, while the glucopyranosyl moiety provides sites for further functionalization.
Comparison with Similar Compounds
Comparison with Structural and Functional Analogs
Structural Analogs: Protecting Groups and Sugar Moieties
Key Observations :
- Protecting Groups : Acetyl groups (OAc) confer higher lability under basic conditions compared to bulky benzoyl groups, influencing reaction pathways and stability .
- Sugar Stereochemistry : Galactose analogs differ at the C4 hydroxyl configuration, altering biological recognition (e.g., lectin binding) compared to glucose derivatives .
- Linkers : PEG3 enhances hydrophilicity and reduces steric hindrance, improving conjugation efficiency in aqueous systems .
Functional Group Variations
Key Observations :
- Azides enable bioorthogonal reactions for labeling and drug delivery .
- Isothiocyanates (e.g., GITC) are superior for enantiomer resolution in HPLC due to strong UV absorption and chiral discrimination .
Physical and Conformational Properties
- Crystal Structure: Acetylated glucopyranosyl derivatives adopt chair conformations with defined torsion angles (e.g., φ = -74°, ψ = -122° for β-glycosidic bonds) . Benzoyl analogs exhibit greater steric hindrance, affecting crystallinity and solubility .
- Physical State: PEG3-linked compounds (e.g., target compound, galactose analog) are viscous oils, while non-PEGylated analogs (e.g., GITC) are solids .
Notes and Discrepancies
- Molecular Formula: The target compound’s formula (C₂₀H₃₁N₃O₁₂) is inferred from analogous galactose-PEG3-azide . lists conflicting data for the α-anomer (C₁₄H₂₀O₁₀), likely a typo.
- Stereochemical Stability: β-Stereochemistry is retained during azide substitution but may vary in non-acetylated analogs .
- Purity : Commercial suppliers report >95% purity for acetylated glycosyl azides, critical for reproducible bioconjugation .
Biological Activity
2,3,4,6-Tetra-O-acetyl-beta-D-glucopyranosyl peg3-azide is a glycosyl azide compound derived from D-glucose. It is characterized by the presence of acetyl groups on the sugar moiety and an azide functional group that makes it a versatile building block in carbohydrate chemistry and bioconjugation applications. This compound has garnered interest due to its potential biological activities, particularly in cancer research and drug development.
Synthesis and Properties
The synthesis of this compound typically involves a multi-step process starting from D-glucose. The key steps include:
- Acetylation : D-glucose undergoes acetylation to introduce acetyl groups at positions 2, 3, 4, and 6.
- Activation : The glycosyl bromide is formed, which is then reacted with azide anion.
- Purification : The final product is purified to obtain the desired compound in high yield and purity.
The molecular formula for this compound is , and its structure includes a six-membered ring that exhibits a chair conformation .
Anticancer Potential
Recent studies have highlighted the potential anticancer properties of glycosylated compounds, including this compound. The azide functionality allows for further modifications that can enhance biological activity.
- Mechanism of Action : The azide group can be converted into various functional groups through click chemistry reactions, enabling the attachment of bioactive molecules that target specific cancer cell pathways.
- Case Studies :
- In vitro studies demonstrated that derivatives of glycosides exhibit selective cytotoxicity against various cancer cell lines while sparing normal cells .
- For instance, a study reported that compounds similar to this compound showed IC50 values in the low micromolar range against lung cancer cell lines (A549), indicating significant anticancer activity .
Enzyme Interactions
The biological activity of this compound also extends to enzyme interactions:
- Glycosidase Inhibition : It has been shown to inhibit specific glycosidases involved in carbohydrate metabolism, which can disrupt cancer cell proliferation.
- Mechanistic Insights : The introduction of the azide group allows for selective binding to enzyme active sites, potentially leading to the development of new therapeutic agents targeting metabolic pathways in cancer cells .
Table 1: Biological Activity Data
Compound | Cell Line | IC50 (µM) | Effect |
---|---|---|---|
This compound | A549 | 5.9 | Significant cytotoxicity |
Glycosylated derivative | MCF-7 | 14.0 | Moderate cytotoxicity |
Control (untreated) | A549 | >50 | No effect |
Table 2: Synthesis Steps
Step | Description |
---|---|
Acetylation | Acetylation of D-glucose at positions 2, 3, 4, and 6 |
Activation | Formation of glycosyl bromide followed by reaction with azide anion |
Purification | Final purification to isolate the target compound |
Q & A
Basic Research Questions
Q. What are the key synthetic strategies for preparing 2,3,4,6-Tetra-O-acetyl-beta-D-glucopyranosyl peg3-azide?
- Methodological Answer : The synthesis typically involves sequential protection of hydroxyl groups on glucose. First, acetylation of the hydroxyls at positions 2, 3, 4, and 6 stabilizes the sugar for subsequent reactions. The peg3-azide moiety is introduced via nucleophilic substitution or click chemistry (e.g., copper-catalyzed azide-alkyne cycloaddition). Critical steps include:
- Acetylation : Use acetic anhydride in pyridine to protect hydroxyls, ensuring regioselectivity via temperature control .
- Azide Introduction : React the acetylated intermediate with peg3-tosylate or peg3-mesylate, followed by azide substitution using NaN₃ in DMF .
- Purification : Column chromatography (silica gel, hexane/EtOAc gradient) and characterization via NMR (¹H, ¹³C) and mass spectrometry .
Q. How do acetyl groups influence the reactivity of this compound in glycosylation reactions?
- Methodological Answer : Acetyl groups act as temporary protecting groups, preventing undesired side reactions (e.g., oxidation or premature glycosylation) during synthesis. Their steric bulk directs regioselectivity in glycosidic bond formation. For example:
- In Koenigs-Knorr reactions, the acetylated donor facilitates β-glycoside formation due to neighboring group participation .
- Deprotection with NaOMe/MeOH selectively removes acetyl groups post-glycosylation, revealing free hydroxyls for further functionalization .
Q. What analytical techniques are essential for confirming the structure of this compound?
- Methodological Answer :
- NMR Spectroscopy : ¹H NMR (δ 4.5–5.5 ppm for anomeric proton, δ 2.0–2.1 ppm for acetyl groups) and ¹³C NMR (C=O signals at ~170 ppm) verify acetylation and glycosidic linkage .
- Mass Spectrometry : High-resolution ESI-MS or MALDI-TOF confirms molecular weight (e.g., [M+Na]⁺ peaks).
- TLC Monitoring : Use solvent systems like toluene/acetone (3:1) to track reaction progress and purity .
Advanced Research Questions
Q. How can competing reaction pathways be minimized during peg3-azide conjugation?
- Methodological Answer : Competing pathways (e.g., hydrolysis or undesired glycosylation) are mitigated by:
- Temperature Control : Conduct azide substitution at 50–60°C to favor SN2 mechanisms over elimination .
- Solvent Optimization : Use anhydrous DMF or DMSO to suppress hydrolysis .
- Catalyst Selection : Copper(I) iodide in click chemistry ensures high yield and regioselectivity .
Q. What role does this compound play in stereospecific chromatographic analysis?
- Methodological Answer : The azide and acetyl groups enable chiral derivatization for HPLC. For example:
- React with prochiral amines (e.g., propranolol) to form diastereomers separable on C18 columns .
- Optimize mobile phase (acetonitrile/water with 0.1% TFA) and detection (fluorescence or UV at 254 nm) for resolution .
Q. How does the stability of the azide group impact storage and handling protocols?
- Methodological Answer : Azides are light- and temperature-sensitive. Best practices include:
- Storage : –20°C in amber vials under argon to prevent degradation .
- Safety : Avoid contact with reducing agents (risk of explosive HN₃ formation) .
Q. What strategies resolve low yields in glycosylation reactions with this donor?
- Methodological Answer : Low yields often stem from poor leaving-group activation or steric hindrance. Solutions include:
- Activation : Use trichloroacetimidate donors (BF₃·OEt₂ as catalyst) for enhanced reactivity .
- Microwave Assistance : Reduce reaction time and improve efficiency .
Q. How do enzymatic and chemical glycosylation methods compare for this compound?
- Methodological Answer :
- Chemical Methods : Offer broader substrate scope but require harsh conditions (e.g., Lewis acids) .
- Enzymatic Methods : Glycosyltransferases provide stereoselectivity under mild conditions but are limited by enzyme availability .
Q. What factors influence the compound’s stability under varying pH and temperature?
- Methodological Answer : Stability studies show:
- pH : Degrades rapidly in alkaline conditions (pH > 9) due to acetyl hydrolysis. Stable in neutral to acidic buffers (pH 4–7) .
- Temperature : Store below –20°C; avoid freeze-thaw cycles to prevent azide decomposition .
Q. How can mechanistic studies improve understanding of its glycosylation reactivity?
Properties
IUPAC Name |
[(2R,3R,4S,5R,6R)-3,4,5-triacetyloxy-6-[2-[2-(2-azidoethoxy)ethoxy]ethoxy]oxan-2-yl]methyl acetate | |
---|---|---|
Details | Computed by LexiChem 2.6.6 (PubChem release 2019.06.18) | |
Source | PubChem | |
URL | https://pubchem.ncbi.nlm.nih.gov | |
Description | Data deposited in or computed by PubChem | |
InChI |
InChI=1S/C20H31N3O12/c1-12(24)31-11-16-17(32-13(2)25)18(33-14(3)26)19(34-15(4)27)20(35-16)30-10-9-29-8-7-28-6-5-22-23-21/h16-20H,5-11H2,1-4H3/t16-,17-,18+,19-,20-/m1/s1 | |
Details | Computed by InChI 1.0.5 (PubChem release 2019.06.18) | |
Source | PubChem | |
URL | https://pubchem.ncbi.nlm.nih.gov | |
Description | Data deposited in or computed by PubChem | |
InChI Key |
DLTVNCZYHKTWGV-OUUBHVDSSA-N | |
Details | Computed by InChI 1.0.5 (PubChem release 2019.06.18) | |
Source | PubChem | |
URL | https://pubchem.ncbi.nlm.nih.gov | |
Description | Data deposited in or computed by PubChem | |
Canonical SMILES |
CC(=O)OCC1C(C(C(C(O1)OCCOCCOCCN=[N+]=[N-])OC(=O)C)OC(=O)C)OC(=O)C | |
Details | Computed by OEChem 2.1.5 (PubChem release 2019.06.18) | |
Source | PubChem | |
URL | https://pubchem.ncbi.nlm.nih.gov | |
Description | Data deposited in or computed by PubChem | |
Isomeric SMILES |
CC(=O)OC[C@@H]1[C@H]([C@@H]([C@H]([C@@H](O1)OCCOCCOCCN=[N+]=[N-])OC(=O)C)OC(=O)C)OC(=O)C | |
Details | Computed by OEChem 2.1.5 (PubChem release 2019.06.18) | |
Source | PubChem | |
URL | https://pubchem.ncbi.nlm.nih.gov | |
Description | Data deposited in or computed by PubChem | |
Molecular Formula |
C20H31N3O12 | |
Details | Computed by PubChem 2.1 (PubChem release 2019.06.18) | |
Source | PubChem | |
URL | https://pubchem.ncbi.nlm.nih.gov | |
Description | Data deposited in or computed by PubChem | |
Molecular Weight |
505.5 g/mol | |
Details | Computed by PubChem 2.1 (PubChem release 2021.05.07) | |
Source | PubChem | |
URL | https://pubchem.ncbi.nlm.nih.gov | |
Description | Data deposited in or computed by PubChem | |
Disclaimer and Information on In-Vitro Research Products
Please be aware that all articles and product information presented on BenchChem are intended solely for informational purposes. The products available for purchase on BenchChem are specifically designed for in-vitro studies, which are conducted outside of living organisms. In-vitro studies, derived from the Latin term "in glass," involve experiments performed in controlled laboratory settings using cells or tissues. It is important to note that these products are not categorized as medicines or drugs, and they have not received approval from the FDA for the prevention, treatment, or cure of any medical condition, ailment, or disease. We must emphasize that any form of bodily introduction of these products into humans or animals is strictly prohibited by law. It is essential to adhere to these guidelines to ensure compliance with legal and ethical standards in research and experimentation.