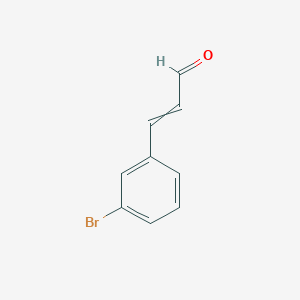
3-(3-Bromophenyl)-2-propenal
Overview
Description
3-(3-Bromophenyl)-2-propenal is a useful research compound. Its molecular formula is C9H7BrO and its molecular weight is 211.05 g/mol. The purity is usually 95%.
BenchChem offers high-quality 3-(3-Bromophenyl)-2-propenal suitable for many research applications. Different packaging options are available to accommodate customers' requirements. Please inquire for more information about 3-(3-Bromophenyl)-2-propenal including the price, delivery time, and more detailed information at info@benchchem.com.
Scientific Research Applications
Antiparasitic Activity : A derivative of 3-(3-Bromophenyl)-2-propenal showed remarkable activity against Trypanosoma cruzi infection, suggesting its potential as a chemotherapeutic agent for Chagas' disease (Pereira, Castro, & Durán, 1998).
Anti-inflammatory and Gastroprotective Properties : Certain chalcones derived from 3-(3-Bromophenyl)-2-propenal demonstrated significant anti-inflammatory and anti-ulcer activities in animal studies (Okunrobo, Usifoh, & Uwaya, 2006).
Anticancer Activities : A novel bromophenol derivative, containing a moiety of 3-(3-Bromophenyl)-2-propenal, was found to have significant anticancer activities on human lung cancer cell lines. It induced cell cycle arrest and apoptosis via the ROS-mediated PI3K/Akt and MAPK signaling pathway (Guo et al., 2018).
Enzyme Inhibition : A study reported the inhibitory effects of a compound related to 3-(3-Bromophenyl)-2-propenal on dopamine beta-hydroxylase, an enzyme involved in the synthesis of norepinephrine, suggesting potential neurological applications (Colombo, Rajashekhar, Giedroc, & Villafranca, 1984).
Chemical Synthesis : The compound has been utilized in chemical synthesis processes, such as the acetoxyallylation of aldehydes mediated by indium, demonstrating its utility in organic chemistry (Lombardo, Girotti, Morganti, & Trombini, 2001).
Biomedical Imaging : Derivatives of 3-(3-Bromophenyl)-2-propenal have been used as ratiometric fluorescent pH probes for strong-acidity pH detection in living cells, indicating their application in biomedical imaging (Nan et al., 2015).
Immunomodulatory Effects : 3-Phenyl-propenal, a principal compound isolated from traditional Chinese medicine and related to 3-(3-Bromophenyl)-2-propenal, has shown effects on the expression of toll-like receptors and downstream signaling components on murine macrophages (Zhao et al., 2008).
properties
IUPAC Name |
3-(3-bromophenyl)prop-2-enal | |
---|---|---|
Details | Computed by LexiChem 2.6.6 (PubChem release 2019.06.18) | |
Source | PubChem | |
URL | https://pubchem.ncbi.nlm.nih.gov | |
Description | Data deposited in or computed by PubChem | |
InChI |
InChI=1S/C9H7BrO/c10-9-5-1-3-8(7-9)4-2-6-11/h1-7H | |
Details | Computed by InChI 1.0.5 (PubChem release 2019.06.18) | |
Source | PubChem | |
URL | https://pubchem.ncbi.nlm.nih.gov | |
Description | Data deposited in or computed by PubChem | |
InChI Key |
QICJGJJHIQBWJR-UHFFFAOYSA-N | |
Details | Computed by InChI 1.0.5 (PubChem release 2019.06.18) | |
Source | PubChem | |
URL | https://pubchem.ncbi.nlm.nih.gov | |
Description | Data deposited in or computed by PubChem | |
Canonical SMILES |
C1=CC(=CC(=C1)Br)C=CC=O | |
Details | Computed by OEChem 2.1.5 (PubChem release 2019.06.18) | |
Source | PubChem | |
URL | https://pubchem.ncbi.nlm.nih.gov | |
Description | Data deposited in or computed by PubChem | |
Molecular Formula |
C9H7BrO | |
Details | Computed by PubChem 2.1 (PubChem release 2019.06.18) | |
Source | PubChem | |
URL | https://pubchem.ncbi.nlm.nih.gov | |
Description | Data deposited in or computed by PubChem | |
Molecular Weight |
211.05 g/mol | |
Details | Computed by PubChem 2.1 (PubChem release 2021.05.07) | |
Source | PubChem | |
URL | https://pubchem.ncbi.nlm.nih.gov | |
Description | Data deposited in or computed by PubChem | |
Product Name |
3-(3-Bromophenyl)-2-propenal |
Retrosynthesis Analysis
AI-Powered Synthesis Planning: Our tool employs the Template_relevance Pistachio, Template_relevance Bkms_metabolic, Template_relevance Pistachio_ringbreaker, Template_relevance Reaxys, Template_relevance Reaxys_biocatalysis model, leveraging a vast database of chemical reactions to predict feasible synthetic routes.
One-Step Synthesis Focus: Specifically designed for one-step synthesis, it provides concise and direct routes for your target compounds, streamlining the synthesis process.
Accurate Predictions: Utilizing the extensive PISTACHIO, BKMS_METABOLIC, PISTACHIO_RINGBREAKER, REAXYS, REAXYS_BIOCATALYSIS database, our tool offers high-accuracy predictions, reflecting the latest in chemical research and data.
Strategy Settings
Precursor scoring | Relevance Heuristic |
---|---|
Min. plausibility | 0.01 |
Model | Template_relevance |
Template Set | Pistachio/Bkms_metabolic/Pistachio_ringbreaker/Reaxys/Reaxys_biocatalysis |
Top-N result to add to graph | 6 |
Feasible Synthetic Routes
Disclaimer and Information on In-Vitro Research Products
Please be aware that all articles and product information presented on BenchChem are intended solely for informational purposes. The products available for purchase on BenchChem are specifically designed for in-vitro studies, which are conducted outside of living organisms. In-vitro studies, derived from the Latin term "in glass," involve experiments performed in controlled laboratory settings using cells or tissues. It is important to note that these products are not categorized as medicines or drugs, and they have not received approval from the FDA for the prevention, treatment, or cure of any medical condition, ailment, or disease. We must emphasize that any form of bodily introduction of these products into humans or animals is strictly prohibited by law. It is essential to adhere to these guidelines to ensure compliance with legal and ethical standards in research and experimentation.