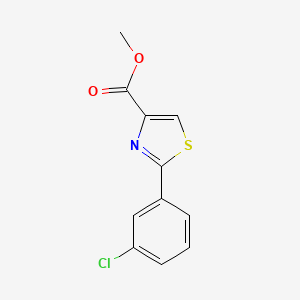
Methyl 2-(3-chlorophenyl)thiazole-4-carboxylate
- Click on QUICK INQUIRY to receive a quote from our team of experts.
- With the quality product at a COMPETITIVE price, you can focus more on your research.
Overview
Description
Methyl 2-(3-chlorophenyl)thiazole-4-carboxylate is a chemical compound that belongs to the thiazole family Thiazoles are heterocyclic compounds containing both sulfur and nitrogen atoms in a five-membered ring This particular compound is characterized by the presence of a methyl ester group at the 4-position of the thiazole ring and a 3-chlorophenyl group at the 2-position
Preparation Methods
Synthetic Routes and Reaction Conditions
The synthesis of Methyl 2-(3-chlorophenyl)thiazole-4-carboxylate typically involves the condensation of 3-chlorobenzaldehyde with thiosemicarbazide to form the corresponding thiosemicarbazone. This intermediate is then cyclized using a suitable dehydrating agent, such as phosphorus oxychloride (POCl3), to yield the thiazole ring. The final step involves esterification with methanol in the presence of an acid catalyst to form the methyl ester.
Industrial Production Methods
Industrial production of this compound may involve similar synthetic routes but on a larger scale. The use of continuous flow reactors and automated systems can enhance the efficiency and yield of the synthesis. Additionally, purification techniques such as recrystallization and chromatography are employed to obtain the compound in high purity.
Chemical Reactions Analysis
Types of Reactions
Methyl 2-(3-chlorophenyl)thiazole-4-carboxylate can undergo various chemical reactions, including:
Oxidation: The thiazole ring can be oxidized using oxidizing agents like hydrogen peroxide or potassium permanganate.
Reduction: The compound can be reduced using reducing agents such as lithium aluminum hydride (LiAlH4).
Substitution: The chlorophenyl group can undergo nucleophilic substitution reactions with nucleophiles like amines or thiols.
Common Reagents and Conditions
Oxidation: Hydrogen peroxide (H2O2) or potassium permanganate (KMnO4) in acidic or basic conditions.
Reduction: Lithium aluminum hydride (LiAlH4) in anhydrous ether.
Substitution: Nucleophiles such as amines or thiols in the presence of a base like sodium hydroxide (NaOH).
Major Products Formed
Oxidation: Oxidized derivatives of the thiazole ring.
Reduction: Reduced forms of the ester group or the thiazole ring.
Substitution: Substituted derivatives with various functional groups replacing the chlorine atom.
Scientific Research Applications
Methyl 2-(3-chlorophenyl)thiazole-4-carboxylate has several scientific research applications:
Chemistry: Used as a building block in the synthesis of more complex thiazole derivatives.
Biology: Investigated for its potential biological activities, including antimicrobial and anticancer properties.
Medicine: Explored as a potential drug candidate due to its ability to interact with biological targets.
Mechanism of Action
The mechanism of action of Methyl 2-(3-chlorophenyl)thiazole-4-carboxylate involves its interaction with specific molecular targets. The compound can bind to enzymes or receptors, modulating their activity. For example, it may inhibit the activity of certain enzymes by binding to their active sites, thereby preventing substrate binding and subsequent catalytic activity. The exact molecular pathways involved depend on the specific biological target and the context of its application .
Comparison with Similar Compounds
Similar Compounds
Methyl 2-(3-bromophenyl)-1,3-thiazole-4-carboxylate: Similar structure with a bromine atom instead of chlorine.
Methyl 2-(3-fluorophenyl)-1,3-thiazole-4-carboxylate: Similar structure with a fluorine atom instead of chlorine.
Methyl 2-(3-methylphenyl)-1,3-thiazole-4-carboxylate: Similar structure with a methyl group instead of chlorine.
Uniqueness
Methyl 2-(3-chlorophenyl)thiazole-4-carboxylate is unique due to the presence of the chlorine atom, which can influence its reactivity and biological activity. The chlorine atom can participate in various interactions, such as hydrogen bonding and halogen bonding, which can affect the compound’s binding affinity to biological targets and its overall pharmacological profile .
Properties
IUPAC Name |
methyl 2-(3-chlorophenyl)-1,3-thiazole-4-carboxylate |
Source
|
---|---|---|
Details | Computed by Lexichem TK 2.7.0 (PubChem release 2021.05.07) | |
Source | PubChem | |
URL | https://pubchem.ncbi.nlm.nih.gov | |
Description | Data deposited in or computed by PubChem | |
InChI |
InChI=1S/C11H8ClNO2S/c1-15-11(14)9-6-16-10(13-9)7-3-2-4-8(12)5-7/h2-6H,1H3 |
Source
|
Details | Computed by InChI 1.0.6 (PubChem release 2021.05.07) | |
Source | PubChem | |
URL | https://pubchem.ncbi.nlm.nih.gov | |
Description | Data deposited in or computed by PubChem | |
InChI Key |
YUJUSIJXJHHHMV-UHFFFAOYSA-N |
Source
|
Details | Computed by InChI 1.0.6 (PubChem release 2021.05.07) | |
Source | PubChem | |
URL | https://pubchem.ncbi.nlm.nih.gov | |
Description | Data deposited in or computed by PubChem | |
Canonical SMILES |
COC(=O)C1=CSC(=N1)C2=CC(=CC=C2)Cl |
Source
|
Details | Computed by OEChem 2.3.0 (PubChem release 2021.05.07) | |
Source | PubChem | |
URL | https://pubchem.ncbi.nlm.nih.gov | |
Description | Data deposited in or computed by PubChem | |
Molecular Formula |
C11H8ClNO2S |
Source
|
Details | Computed by PubChem 2.1 (PubChem release 2021.05.07) | |
Source | PubChem | |
URL | https://pubchem.ncbi.nlm.nih.gov | |
Description | Data deposited in or computed by PubChem | |
Molecular Weight |
253.71 g/mol |
Source
|
Details | Computed by PubChem 2.1 (PubChem release 2021.05.07) | |
Source | PubChem | |
URL | https://pubchem.ncbi.nlm.nih.gov | |
Description | Data deposited in or computed by PubChem | |
Disclaimer and Information on In-Vitro Research Products
Please be aware that all articles and product information presented on BenchChem are intended solely for informational purposes. The products available for purchase on BenchChem are specifically designed for in-vitro studies, which are conducted outside of living organisms. In-vitro studies, derived from the Latin term "in glass," involve experiments performed in controlled laboratory settings using cells or tissues. It is important to note that these products are not categorized as medicines or drugs, and they have not received approval from the FDA for the prevention, treatment, or cure of any medical condition, ailment, or disease. We must emphasize that any form of bodily introduction of these products into humans or animals is strictly prohibited by law. It is essential to adhere to these guidelines to ensure compliance with legal and ethical standards in research and experimentation.