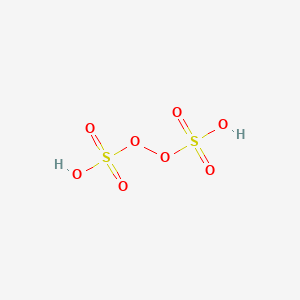
Peroxydisulfuric acid
Overview
Description
Peroxydisulfuric acid, also known as Marshall’s acid, is an inorganic compound with the chemical formula H₂S₂O₈. It is a powerful oxidizing agent and is characterized by the presence of a peroxide group. This compound was first discovered by Professor Hugh Marshall in 1891 . This compound is a colorless solid that decomposes at temperatures above 65°C .
Preparation Methods
Synthetic Routes and Reaction Conditions: Peroxydisulfuric acid can be synthesized through several methods:
Reaction of Chlorosulfuric Acid with Hydrogen Peroxide: This method involves the reaction of chlorosulfuric acid (ClSO₃H) with hydrogen peroxide (H₂O₂) to produce this compound and hydrochloric acid (HCl) as a byproduct. [ 2 \text{ClSO}_3\text{H} + \text{H}_2\text{O}_2 \rightarrow \text{H}_2\text{S}_2\text{O}_8 + 2 \text{HCl} ]
Electrolysis of Sulfuric Acid: Another method involves the electrolysis of moderately concentrated sulfuric acid (60-70%) using platinum electrodes at high current density and voltage. [ 2 \text{HSO}_4^- \rightarrow \text{H}_2\text{S}_2\text{O}_8 + 2 \text{e}^- ]
Industrial Production Methods: In industrial settings, this compound is often produced by the electrolysis of sulfuric acid solutions. This method is preferred due to its efficiency and scalability .
Chemical Reactions Analysis
Peroxydisulfuric acid undergoes various types of chemical reactions, including:
Oxidation Reactions: It acts as a strong oxidizing agent.
Decomposition: In water, this compound decomposes to form hydrogen peroxide (H₂O₂) and sulfuric acid (H₂SO₄). [ \text{H}_2\text{S}_2\text{O}_8 + 2 \text{H}_2\text{O} \rightarrow \text{H}_2\text{O}_2 + 2 \text{H}_2\text{SO}_4 ]
Scientific Research Applications
Peroxydisulfuric acid has a wide range of applications in scientific research and industry:
Chemistry: It is used as a precursor to various salts, such as sodium peroxydisulfate, potassium peroxydisulfate, and ammonium peroxydisulfate.
Biology and Medicine: Due to its strong oxidizing properties, this compound is used in the synthesis of disinfectants and bleaching agents.
Industry: It is utilized in the production of detergents and as a laboratory reagent.
Mechanism of Action
The mechanism of action of peroxydisulfuric acid primarily involves its strong oxidizing properties. The peroxide group in its structure allows it to release oxygen, which can then participate in various oxidation reactions. This makes it effective in breaking down organic compounds and initiating polymerization reactions .
Comparison with Similar Compounds
Peroxydisulfuric acid can be compared with other similar compounds, such as:
Peroxymonosulfuric Acid (H₂SO₅):
Pyrosulfuric Acid (H₂S₂O₇): This compound does not contain a peroxide group and is primarily used in the production of sulfuric acid.
This compound stands out due to its strong oxidizing properties and stability compared to other peroxy acids .
Properties
IUPAC Name |
sulfooxy hydrogen sulfate | |
---|---|---|
Source | PubChem | |
URL | https://pubchem.ncbi.nlm.nih.gov | |
Description | Data deposited in or computed by PubChem | |
InChI |
InChI=1S/H2O8S2/c1-9(2,3)7-8-10(4,5)6/h(H,1,2,3)(H,4,5,6) | |
Source | PubChem | |
URL | https://pubchem.ncbi.nlm.nih.gov | |
Description | Data deposited in or computed by PubChem | |
InChI Key |
JRKICGRDRMAZLK-UHFFFAOYSA-N | |
Source | PubChem | |
URL | https://pubchem.ncbi.nlm.nih.gov | |
Description | Data deposited in or computed by PubChem | |
Canonical SMILES |
OS(=O)(=O)OOS(=O)(=O)O | |
Source | PubChem | |
URL | https://pubchem.ncbi.nlm.nih.gov | |
Description | Data deposited in or computed by PubChem | |
Molecular Formula |
H2S2O8, H2O8S2 | |
Record name | peroxydisulfuric acid | |
Source | Wikipedia | |
URL | https://en.wikipedia.org/wiki/Peroxydisulfuric_acid | |
Description | Chemical information link to Wikipedia. | |
Source | PubChem | |
URL | https://pubchem.ncbi.nlm.nih.gov | |
Description | Data deposited in or computed by PubChem | |
DSSTOX Substance ID |
DTXSID00894926 | |
Record name | Peroxodisulfuric acid | |
Source | EPA DSSTox | |
URL | https://comptox.epa.gov/dashboard/DTXSID00894926 | |
Description | DSSTox provides a high quality public chemistry resource for supporting improved predictive toxicology. | |
Molecular Weight |
194.15 g/mol | |
Source | PubChem | |
URL | https://pubchem.ncbi.nlm.nih.gov | |
Description | Data deposited in or computed by PubChem | |
CAS No. |
13445-49-3 | |
Record name | Peroxydisulfuric acid | |
Source | CAS Common Chemistry | |
URL | https://commonchemistry.cas.org/detail?cas_rn=13445-49-3 | |
Description | CAS Common Chemistry is an open community resource for accessing chemical information. Nearly 500,000 chemical substances from CAS REGISTRY cover areas of community interest, including common and frequently regulated chemicals, and those relevant to high school and undergraduate chemistry classes. This chemical information, curated by our expert scientists, is provided in alignment with our mission as a division of the American Chemical Society. | |
Explanation | The data from CAS Common Chemistry is provided under a CC-BY-NC 4.0 license, unless otherwise stated. | |
Record name | Peroxydisulfuric acid | |
Source | ChemIDplus | |
URL | https://pubchem.ncbi.nlm.nih.gov/substance/?source=chemidplus&sourceid=0013445493 | |
Description | ChemIDplus is a free, web search system that provides access to the structure and nomenclature authority files used for the identification of chemical substances cited in National Library of Medicine (NLM) databases, including the TOXNET system. | |
Record name | Peroxydisulfuric acid ([(HO)S(O)2]2O2) | |
Source | EPA Chemicals under the TSCA | |
URL | https://www.epa.gov/chemicals-under-tsca | |
Description | EPA Chemicals under the Toxic Substances Control Act (TSCA) collection contains information on chemicals and their regulations under TSCA, including non-confidential content from the TSCA Chemical Substance Inventory and Chemical Data Reporting. | |
Record name | Peroxodisulfuric acid | |
Source | EPA DSSTox | |
URL | https://comptox.epa.gov/dashboard/DTXSID00894926 | |
Description | DSSTox provides a high quality public chemistry resource for supporting improved predictive toxicology. | |
Record name | PEROXYDISULFURIC ACID | |
Source | FDA Global Substance Registration System (GSRS) | |
URL | https://gsrs.ncats.nih.gov/ginas/app/beta/substances/2RQ1860626 | |
Description | The FDA Global Substance Registration System (GSRS) enables the efficient and accurate exchange of information on what substances are in regulated products. Instead of relying on names, which vary across regulatory domains, countries, and regions, the GSRS knowledge base makes it possible for substances to be defined by standardized, scientific descriptions. | |
Explanation | Unless otherwise noted, the contents of the FDA website (www.fda.gov), both text and graphics, are not copyrighted. They are in the public domain and may be republished, reprinted and otherwise used freely by anyone without the need to obtain permission from FDA. Credit to the U.S. Food and Drug Administration as the source is appreciated but not required. | |
Synthesis routes and methods I
Procedure details
Synthesis routes and methods II
Procedure details
Retrosynthesis Analysis
AI-Powered Synthesis Planning: Our tool employs the Template_relevance Pistachio, Template_relevance Bkms_metabolic, Template_relevance Pistachio_ringbreaker, Template_relevance Reaxys, Template_relevance Reaxys_biocatalysis model, leveraging a vast database of chemical reactions to predict feasible synthetic routes.
One-Step Synthesis Focus: Specifically designed for one-step synthesis, it provides concise and direct routes for your target compounds, streamlining the synthesis process.
Accurate Predictions: Utilizing the extensive PISTACHIO, BKMS_METABOLIC, PISTACHIO_RINGBREAKER, REAXYS, REAXYS_BIOCATALYSIS database, our tool offers high-accuracy predictions, reflecting the latest in chemical research and data.
Strategy Settings
Precursor scoring | Relevance Heuristic |
---|---|
Min. plausibility | 0.01 |
Model | Template_relevance |
Template Set | Pistachio/Bkms_metabolic/Pistachio_ringbreaker/Reaxys/Reaxys_biocatalysis |
Top-N result to add to graph | 6 |
Feasible Synthetic Routes
Disclaimer and Information on In-Vitro Research Products
Please be aware that all articles and product information presented on BenchChem are intended solely for informational purposes. The products available for purchase on BenchChem are specifically designed for in-vitro studies, which are conducted outside of living organisms. In-vitro studies, derived from the Latin term "in glass," involve experiments performed in controlled laboratory settings using cells or tissues. It is important to note that these products are not categorized as medicines or drugs, and they have not received approval from the FDA for the prevention, treatment, or cure of any medical condition, ailment, or disease. We must emphasize that any form of bodily introduction of these products into humans or animals is strictly prohibited by law. It is essential to adhere to these guidelines to ensure compliance with legal and ethical standards in research and experimentation.