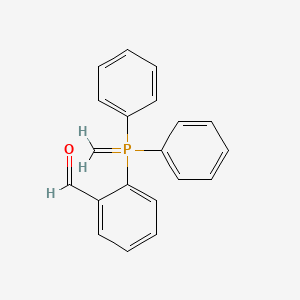
Formyl methylenetriphenylphosphorane
Overview
Description
Formyl methylenetriphenylphosphorane is a useful research compound. Its molecular formula is C20H17OP and its molecular weight is 304.3 g/mol. The purity is usually 95%.
BenchChem offers high-quality this compound suitable for many research applications. Different packaging options are available to accommodate customers' requirements. Please inquire for more information about this compound including the price, delivery time, and more detailed information at info@benchchem.com.
Scientific Research Applications
Alemagna et al. (1985) reported that o.formylarylazomethylenetriphenylphosphoranes undergo thermal intramolecular cyclization to produce 3-oxo-indazolin-2-yl-methylenetriphenylphosphorane derivatives, which are then rearranged to 4-oxo-l,4-dihydroquinazoline derivatives. This study indicates a synthetic application of this compound in producing specific chemical structures (Alemagna, Buttero, Licandro, Maiorana, & Papagni, 1985).
Mátyus et al. (1991) demonstrated the reaction of ethoxycarbonylmethylenetriphenylphosphorane with 5-formyl-1,3,6-trimethyluracil, leading to the formation of acrylate and a novel phosphorus ylide/phosphorane. This research contributes to understanding the chemistry of uracils and their interaction with phosphoranes (Mátyus, Nieger, & Wamhoff, 1991).
Macomber and Rausch (1983) explored the preparation of functionally substituted cyclopentadienyltricarbonylmethyl derivatives of chromium, molybdenum, and tungsten using methylenetriphenylphosphorane. The study shows the compound's role in producing these metal derivatives, important in organometallic chemistry (Macomber & Rausch, 1983).
Kumar and Bodas (2000) used (trimethylsilyl)methylenetriphenylphosphorane in the synthesis of 4H-chromen-4-ones via intramolecular Wittig reaction. This indicates its use in creating specific organic compounds, particularly in pharmaceutical and synthetic organic chemistry (Kumar & Bodas, 2000).
Bart (1969) elucidated the structure of methylenetriphenylphosphorane, providing foundational information about its molecular configuration. This structural analysis is vital for understanding its reactivity and applications in various chemical reactions (Bart, 1969).
properties
IUPAC Name |
2-[methylidene(diphenyl)-λ5-phosphanyl]benzaldehyde | |
---|---|---|
Details | Computed by Lexichem TK 2.7.0 (PubChem release 2021.05.07) | |
Source | PubChem | |
URL | https://pubchem.ncbi.nlm.nih.gov | |
Description | Data deposited in or computed by PubChem | |
InChI |
InChI=1S/C20H17OP/c1-22(18-11-4-2-5-12-18,19-13-6-3-7-14-19)20-15-9-8-10-17(20)16-21/h2-16H,1H2 | |
Details | Computed by InChI 1.0.6 (PubChem release 2021.05.07) | |
Source | PubChem | |
URL | https://pubchem.ncbi.nlm.nih.gov | |
Description | Data deposited in or computed by PubChem | |
InChI Key |
WLNHKVMQWPCCER-UHFFFAOYSA-N | |
Details | Computed by InChI 1.0.6 (PubChem release 2021.05.07) | |
Source | PubChem | |
URL | https://pubchem.ncbi.nlm.nih.gov | |
Description | Data deposited in or computed by PubChem | |
Canonical SMILES |
C=P(C1=CC=CC=C1)(C2=CC=CC=C2)C3=CC=CC=C3C=O | |
Details | Computed by OEChem 2.3.0 (PubChem release 2021.05.07) | |
Source | PubChem | |
URL | https://pubchem.ncbi.nlm.nih.gov | |
Description | Data deposited in or computed by PubChem | |
Molecular Formula |
C20H17OP | |
Details | Computed by PubChem 2.1 (PubChem release 2021.05.07) | |
Source | PubChem | |
URL | https://pubchem.ncbi.nlm.nih.gov | |
Description | Data deposited in or computed by PubChem | |
Molecular Weight |
304.3 g/mol | |
Details | Computed by PubChem 2.1 (PubChem release 2021.05.07) | |
Source | PubChem | |
URL | https://pubchem.ncbi.nlm.nih.gov | |
Description | Data deposited in or computed by PubChem | |
Retrosynthesis Analysis
AI-Powered Synthesis Planning: Our tool employs the Template_relevance Pistachio, Template_relevance Bkms_metabolic, Template_relevance Pistachio_ringbreaker, Template_relevance Reaxys, Template_relevance Reaxys_biocatalysis model, leveraging a vast database of chemical reactions to predict feasible synthetic routes.
One-Step Synthesis Focus: Specifically designed for one-step synthesis, it provides concise and direct routes for your target compounds, streamlining the synthesis process.
Accurate Predictions: Utilizing the extensive PISTACHIO, BKMS_METABOLIC, PISTACHIO_RINGBREAKER, REAXYS, REAXYS_BIOCATALYSIS database, our tool offers high-accuracy predictions, reflecting the latest in chemical research and data.
Strategy Settings
Precursor scoring | Relevance Heuristic |
---|---|
Min. plausibility | 0.01 |
Model | Template_relevance |
Template Set | Pistachio/Bkms_metabolic/Pistachio_ringbreaker/Reaxys/Reaxys_biocatalysis |
Top-N result to add to graph | 6 |
Feasible Synthetic Routes
Disclaimer and Information on In-Vitro Research Products
Please be aware that all articles and product information presented on BenchChem are intended solely for informational purposes. The products available for purchase on BenchChem are specifically designed for in-vitro studies, which are conducted outside of living organisms. In-vitro studies, derived from the Latin term "in glass," involve experiments performed in controlled laboratory settings using cells or tissues. It is important to note that these products are not categorized as medicines or drugs, and they have not received approval from the FDA for the prevention, treatment, or cure of any medical condition, ailment, or disease. We must emphasize that any form of bodily introduction of these products into humans or animals is strictly prohibited by law. It is essential to adhere to these guidelines to ensure compliance with legal and ethical standards in research and experimentation.